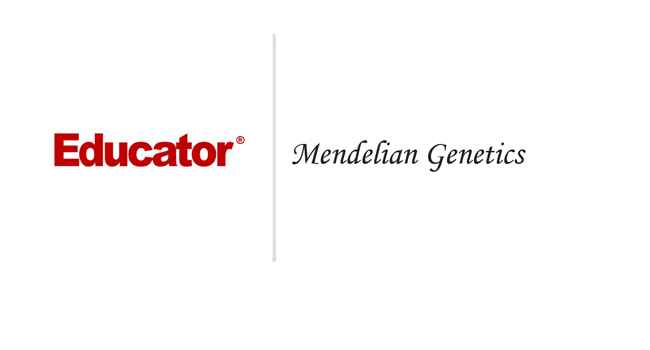
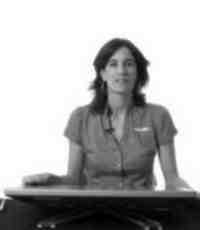
Dr. Carleen Eaton
Mendelian Genetics
Slide Duration:Table of Contents
56m 18s
- Intro0:00
- Elements0:09
- Elements0:48
- Matter0:55
- Naturally Occurring Elements1:12
- Atomic Number and Atomic Mass2:39
- Compounds3:06
- Molecule3:07
- Compounds3:14
- Examples3:20
- Atoms4:53
- Atoms4:56
- Protons, Neutrons, and Electrons5:29
- Isotopes10:42
- Energy Levels of Electrons13:01
- Electron Shells13:13
- Valence Shell13:22
- Example: Electron Shells and Potential Energy13:28
- Covalent Bonds19:52
- Covalent Bonds19:54
- Examples20:03
- Polar and Nonpolar Covalent Bonds23:54
- Polar Bond24:07
- Nonpolar Bonds24:17
- Examples24:25
- Ionic Bonds29:04
- Ionic Bond, Cations, Anions29:19
- Example: NaCl29:30
- Hydrogen Bond33:18
- Hydrogen Bond33:20
- Chemical Reactions35:36
- Example: Reactants, Products and Chemical Reactions35:45
- Molecular Mass and Molar Concentration38:45
- Avogadro's Number and Mol39:12
- Examples: Molecular Mass and Molarity42:10
- Example 1: Proton, Neutrons and Electrons47:05
- Example 2: Reactants and Products49:35
- Example 3: Bonding52:39
- Example 4: Mass53:59
50m 23s
- Intro0:00
- Molecular Structure of Water0:21
- Molecular Structure of Water0:27
- Properties of Water4:30
- Cohesive4:55
- Transpiration5:29
- Adhesion6:20
- Surface Tension7:17
- Properties of Water, cont.9:14
- Specific Heat9:25
- High Heat Capacity13:24
- High Heat of Evaporation16:42
- Water as a Solvent21:13
- Solution21:28
- Solvent21:48
- Example: Water as a Solvent22:22
- Acids and Bases25:40
- Example25:41
- pH36:30
- pH Scale: Acidic, Neutral, and Basic36:35
- Example 1: Molecular Structure and Properties of Water41:18
- Example 2: Special Properties of Water42:53
- Example 3: pH Scale44:46
- Example 4: Acids and Bases46:19
53m 54s
- Intro0:00
- Organic Compounds0:09
- Organic Compounds0:11
- Inorganic Compounds0:15
- Examples: Organic Compounds1:15
- Isomers5:52
- Isomers5:55
- Structural Isomers6:23
- Geometric Isomers8:14
- Enantiomers9:55
- Functional Groups12:46
- Examples: Functional Groups12:59
- Amino Group13:51
- Carboxyl Group14:38
- Hydroxyl Group15:22
- Methyl Group16:14
- Carbonyl Group16:30
- Phosphate Group17:51
- Carbohydrates18:26
- Carbohydrates19:07
- Example: Monosaccharides21:12
- Carbohydrates, cont.24:11
- Disaccharides, Polysaccharides and Examples24:21
- Lipids35:52
- Examples of Lipids36:04
- Saturated and Unsaturated38:57
- Phospholipids43:26
- Phospholipids43:29
- Example43:34
- Steroids46:24
- Cholesterol46:28
- Example 1: Isomers48:11
- Example 2: Functional Groups50:45
- Example 3: Galactose, Ketose, and Aldehyde Sugar52:24
- Example 4: Class of Molecules53:06
37m 23s
- Intro0:00
- Nucleic Acids0:09
- Deoxyribonucleic Acid (DNA) and Ribonucleic Acid (RNA)0:29
- Nucleic Acids, cont.2:56
- Purines3:10
- Pyrimidines3:32
- Double Helix4:59
- Double Helix and Example5:01
- Proteins12:33
- Amino Acids and Polypeptides12:39
- Examples: Amino Acid13:25
- Polypeptide Formation18:09
- Peptide Bonds18:14
- Primary Structure18:35
- Protein Structure23:19
- Secondary Structure23:22
- Alpha Helices and Beta Pleated Sheets23:34
- Protein Structure25:43
- Tertiary Structure25:44
- 5 Types of Interaction26:56
- Example 1: Complementary DNA Strand31:45
- Example 2: Differences Between DNA and RNA33:19
- Example 3: Amino Acids34:32
- Example 4: Tertiary Structure of Protein35:46
45m 50s
- Intro0:00
- Cell Theory and Cell Types0:12
- Cell Theory0:13
- Prokaryotic and Eukaryotic Cells0:36
- Endosymbiotic Theory1:13
- Study of Cells4:07
- Tools and Techniques4:08
- Light Microscopes5:08
- Light vs. Electron Microscopes: Magnification5:18
- Light vs. Electron Microscopes: Resolution6:26
- Light vs. Electron Microscopes: Specimens7:53
- Electron Microscopes: Transmission and Scanning8:28
- Cell Fractionation10:01
- Cell Fractionation Step 1: Homogenization10:33
- Cell Fractionation Step 2: Spin11:24
- Cell Fractionation Step 3: Differential Centrifugation11:53
- Comparison of Prokaryotic and Eukaryotic Cells14:12
- Prokaryotic vs. Eukaryotic Cells: Domains14:43
- Prokaryotic vs. Eukaryotic Cells: Plasma Membrane15:40
- Prokaryotic vs. Eukaryotic Cells: Cell Walls16:15
- Prokaryotic vs. Eukaryotic Cells: Genetic Materials16:38
- Prokaryotic vs. Eukaryotic Cells: Structures17:28
- Prokaryotic vs. Eukaryotic Cells: Unicellular and Multicellular18:19
- Prokaryotic vs. Eukaryotic Cells: Size18:31
- Plasmids18:52
- Prokaryotic vs. Eukaryotic Cells19:22
- Nucleus19:24
- Organelles19:48
- Cytoskeleton20:02
- Cell Wall20:35
- Ribosomes20:57
- Size21:37
- Comparison of Plant and Animal Cells22:15
- Plasma Membrane22:55
- Plant Cells Only: Cell Walls23:12
- Plant Cells Only: Central Vacuole25:08
- Animal Cells Only: Centrioles26:40
- Animal Cells Only: Lysosomes27:43
- Plant vs. Animal Cells29:16
- Overview of Plant and Animal Cells29:17
- Evidence for the Endosymbiotic Theory30:52
- Characteristics of Mitochondria and Chloroplasts30:54
- Example 1: Prokaryotic vs. Eukaryotic Cells35:44
- Example 2: Endosymbiotic Theory and Evidence38:38
- Example 3: Plant and Animal Cells41:49
- Example 4: Cell Fractionation43:44
59m 38s
- Intro0:00
- Prokaryotic Cells0:09
- Shapes of Prokaryotic Cells0:22
- Cell Wall1:19
- Capsule3:23
- Pili/Fimbria3:54
- Flagella4:35
- Nucleoid6:16
- Plasmid6:37
- Ribosomes7:09
- Eukaryotic Cells (Animal Cell Structure)8:01
- Plasma Membrane8:13
- Microvilli8:48
- Nucleus9:47
- Nucleolus11:06
- Ribosomes: Free and Bound12:26
- Rough Endoplasmic Reticulum (RER)13:43
- Eukaryotic Cells (Animal Cell Structure), cont.14:51
- Endoplasmic Reticulum: Smooth and Rough15:08
- Golgi Apparatus17:55
- Vacuole20:43
- Lysosome22:01
- Mitochondria25:40
- Peroxisomes28:18
- Cytoskeleton30:41
- Cytoplasm and Cytosol30:53
- Microtubules: Centrioles, Spindel Fibers, Clagell, Cillia32:06
- Microfilaments36:39
- Intermediate Filaments and Kerotin38:52
- Eukaryotic Cells (Plant Cell Structure)40:08
- Plasma Membrane, Primary Cell Wall, and Secondary Cell Wall40:30
- Middle Lamella43:21
- Central Cauole44:12
- Plastids: Leucoplasts, Chromoplasts, Chrloroplasts45:35
- Chloroplasts47:06
- Example 1: Structures and Functions48:46
- Example 2: Cell Walls51:19
- Example 3: Cytoskeleton52:53
- Example 4: Antibiotics and the Endosymbiosis Theory56:55
53m 10s
- Intro0:00
- Cell Membrane Structure0:09
- Phospholipids Bilayer0:11
- Chemical Structure: Amphipathic and Fatty Acids0:25
- Cell Membrane Proteins2:44
- Fluid Mosaic Model2:45
- Peripheral Proteins and Integral Proteins3:19
- Transmembrane Proteins4:34
- Cholesterol4:48
- Functions of Membrane Proteins6:39
- Transport Across Cell Membranes9:52
- Transport Across Cell Membranes9:53
- Methods of Passive Transport12:07
- Passive and Active Transport12:08
- Simple Diffusion12:45
- Facilitated Diffusion15:20
- Osmosis17:17
- Definition and Example of Osmosis17:18
- Hypertonic, Hypotonic, and Isotonic21:47
- Active Transport27:57
- Active Transport28:17
- Sodium and Potassium Pump29:45
- Cotransport34:38
- 2 Types of Active Transport37:09
- Endocytosis and Exocytosis37:38
- Endocytosis and Exocytosis37:51
- Types of Endocytosis: Pinocytosis40:39
- Types of Endocytosis: Phagocytosis41:02
- Receptor Mediated Endocytosis41:27
- Receptor Mediated Endocytosis41:28
- Example 1: Cell Membrane and Permeable Substances43:59
- Example 2: Osmosis45:20
- Example 3: Active Transport, Cotransport, Simple and Facilitated Diffusion47:36
- Example 4: Match Terms with Definition50:55
57m 9s
- Intro0:00
- Extracellular Matrix0:28
- The Extracellular Matrix (ECM)0:29
- ECM in Animal Cells0:55
- Fibronectin and Integrins1:34
- Intercellular Communication in Plants2:48
- Intercellular Communication in Plants: Plasmodesmata2:50
- Cell to Cell Communication in Animal Cells3:39
- Cell Junctions3:42
- Desmosomes3:54
- Tight Junctions5:07
- Gap Junctions7:00
- Cell Signaling8:17
- Cell Signaling: Ligand and Signal Transduction Pathway8:18
- Direct Contact8:48
- Over Distances Contact and Hormones10:09
- Stages of Cell Signaling11:53
- Reception Phase11:54
- Transduction Phase13:49
- Response Phase14:45
- Cell Membrane Receptors15:37
- G-Protein Coupled Receptor15:38
- Cell Membrane Receptor, Cont.21:37
- Receptor Tyrosine Kinases (RTKs)21:38
- Autophosphorylation, Monomer, and Dimer22:57
- Cell Membrane Receptor, Cont.27:01
- Ligand-Gated Ion Channels27:02
- Intracellular Receptors29:43
- Intracellular Receptor and Receptor -Ligand Complex29:44
- Signal Transduction32:57
- Signal Transduction Pathways32:58
- Adenylyl Cyclase and cAMP35:53
- Second Messengers39:18
- cGMP, Inositol Trisphosphate, and Diacylglycerol39:20
- Cell Response45:15
- Cell Response45:16
- Apoptosis46:57
- Example 1: Tight Junction and Gap Junction48:29
- Example 2: Three Phases of Cell Signaling51:48
- Example 3: Ligands and Binding of Hormone54:03
- Example 4: Signal Transduction56:06
37m 49s
- Intro0:00
- Functions of Cell Division0:09
- Overview of Cell Division: Reproduction, Growth, and Repair0:11
- Important Term: Daughter Cells2:25
- Chromosome Structure3:36
- Chromosome Structure: Sister Chromatids and Centromere3:37
- Chromosome Structure: Chromatin4:31
- Chromosome with One Chromatid or Two Chromatids5:25
- Chromosome Structure: Long and Short Arm6:49
- Mitosis and Meiosis7:00
- Mitosis7:41
- Meiosis8:40
- The Cell Cycle10:43
- Mitotic Phase and Interphase10:44
- Cytokinesis15:51
- Cytokinesis in Animal Cell: Cleavage Furrow15:52
- Cytokinesis in Plant Cell: Cell Plate17:28
- Control of the Cell Cycle18:28
- Cell Cycle Control System and Checkpoints18:29
- Cyclins and Cyclin Dependent Kinases21:18
- Cyclins and Cyclin Dependent Kinases (CDKSs)21:20
- MPF23:17
- Internal Factor Regulating Cell Cycle24:00
- External Factor Regulating Cell Cycle24:53
- Contact Inhibition and Anchorage Dependent25:53
- Cancer and the Cell Cycle27:42
- Cancer Cells27:46
- Example1: Parts of the Chromosome30:15
- Example 2: Cell Cycle31:50
- Example 3: Control of the Cell Cycle33:32
- Example 4: Cancer and the Cell35:01
35m 1s
- Intro0:00
- Review of the Cell Cycle0:09
- Interphase: G1 Phase0:34
- Interphase: S Phase0:56
- Interphase: G2 Phase1:31
- M Phase: Mitosis and Cytokinesis1:47
- Overview of Mitosis3:08
- What is Mitosis?3:10
- Overview of Mitosis3:17
- Diploid and Haploid5:37
- Homologous Chromosomes6:04
- The Spindle Apparatus11:57
- The Spindle Apparatus12:00
- Centrosomes and Centrioles12:40
- Microtubule Organizing Center13:03
- Spindle Fiber of Spindle Microtubules13:23
- Kinetochores14:06
- Asters15:45
- Prophase16:47
- First Phase of Mitosis: Prophase16:54
- Metaphase20:05
- Second Phase of Mitosis: Metaphase20:10
- Anaphase22:52
- Third Phase of Mitosis: Anaphase22:53
- Telophase and Cytokinesis24:34
- Last Phase of Mitosis: Telophase and Cytokinesis24:35
- Summary of Mitosis27:46
- Summary of Mitosis27:47
- Example 1: Spindle Apparatus28:50
- Example 2: Last Phase of Mitosis30:39
- Example 3: Prophase32:41
- Example 4: Identify the Phase33:52
1h 58s
- Intro0:00
- Haploid and Diploid Cells0:09
- Diploid and Somatic Cells0:29
- Haploid and Gametes1:20
- Example: Human Cells and Chromosomes1:41
- Sex Chromosomes6:00
- Comparison of Mitosis and Meiosis10:42
- Mitosis Vs. Meiosis: Cell Division10:59
- Mitosis Vs. Meiosis: Daughter Cells12:31
- Meiosis: Pairing of Homologous Chromosomes13:40
- Mitosis and Meiosis14:21
- Process of Mitosis14:27
- Process of Meiosis16:12
- Synapsis and Crossing Over19:14
- Prophase I: Synapsis and Crossing Over19:15
- Chiasmata22:33
- Meiosis I25:49
- Prophase I: Crossing Over25:50
- Metaphase I: Homologs Line Up26:00
- Anaphase I: Homologs Separate28:16
- Telophase I and Cytokinesis29:15
- Independent Assortment30:58
- Meiosis II32:17
- Propphase II33:50
- Metaphase II34:06
- Anaphase II34:50
- Telophase II36:09
- Cytokinesis37:00
- Summary of Meiosis38:15
- Summary of Meiosis38:16
- Cell Division Mechanism in Plants41:57
- Example 1: Cell Division and Meiosis46:15
- Example 2: Phases of Meiosis50:22
- Example 3: Label the Figure54:29
- Example 4: Four Differences Between Mitosis and Meiosis56:37
51m 3s
- Intro0:00
- Law of Thermodynamics0:08
- Thermodynamics0:09
- The First Law of Thermodynamics0:37
- The Second Law of Thermodynamics1:24
- Entropy1:35
- The Gibbs Free Energy Equation3:07
- The Gibbs Free Energy Equation3:08
- ATP8:23
- Adenosine Triphosphate (ATP)8:24
- Cellular Respiration11:32
- Catabolic Pathways12:28
- Anabolic Pathways12:54
- Enzymes14:31
- Enzymes14:32
- Enzymes and Exergonic Reaction14:40
- Enzymes and Endergonic Reaction16:36
- Enzyme Specificity21:29
- Substrate21:41
- Induced Fit23:04
- Factors Affecting Enzyme Activity25:55
- Substrate Concentration26:07
- pH27:10
- Temperature29:14
- Presence of Cofactors29:57
- Regulation of Enzyme Activity31:12
- Competitive Inhibitors32:13
- Noncompetitive Inhibitors33:52
- Feedback Inhibition35:22
- Allosteric Interactions36:56
- Allosteric Regulators37:00
- Example 1: Is the Inhibitor Competitive or Noncompetitive?40:49
- Example 2: Thermophiles44:18
- Example 3: Exergonic or Endergonic46:09
- Example 4: Energy Vs. Reaction Progress Graph48:47
38m 1s
- Intro0:00
- Cellular Respiration Overview0:13
- Cellular Respiration0:14
- Anaerobic Respiration vs. Aerobic Respiration3:50
- Glycolysis Overview4:48
- Overview of Glycolysis4:50
- Glycolysis Involves a Redox Reaction7:02
- Redox Reaction7:04
- Glycolysis15:04
- Important Facts About Glycolysis15:07
- Energy Invested Phase16:12
- Splitting of Fructose 1,6-Phosphate and Energy Payoff Phase17:50
- Substrate Level Phophorylation22:12
- Aerobic Versus Anaerobic Respiration23:57
- Aerobic Versus Anaerobic Respiration23:58
- Cellular Respiration Overview27:15
- When Cellular Respiration is Anaerobic27:17
- Glycolysis28:26
- Alcohol Fermentation28:45
- Lactic Acid Fermentation29:58
- Example 1: Glycolysis31:04
- Example 2: Glycolysis, Fermentation and Anaerobic Respiration33:44
- Example 3: Aerobic Respiration Vs. Anaerobic Respiration35:25
- Example 4: Exergonic Reaction and Endergonic Reaction36:42
51m 6s
- Intro0:00
- Aerobic Vs. Anaerobic Respiration0:06
- Aerobic and Anaerobic Comparison0:07
- Review of Glycolysis1:48
- Overview of Glycolysis2:06
- Glycolysis: Energy Investment Phase2:25
- Glycolysis: Energy Payoff Phase2:58
- Conversion of Pyruvate to Acetyl CoA4:55
- Conversion of Pyruvate to Acetyl CoA4:56
- Energy Formation8:06
- Mitochondrial Structure8:58
- Endosymbiosis Theory9:23
- Matrix10:00
- Outer Membrane, Inner Membrane, and Intermembrane Space10:43
- Cristae11:47
- The Citric Acid Cycle12:11
- The Citric Acid Cycle (Also Called Krebs Cycle)12:12
- Substrate Level Phosphorylation18:47
- Summary of ATP, NADH, and FADH2 Production23:13
- Process: Glycolysis23:28
- Process: Acetyl CoA Production23:36
- Process: Citric Acid Cycle23:52
- The Electron Transport Chain24:24
- Oxidative Phosphorylation24:28
- The Electron Transport Chain and ATP Synthase25:20
- Carrier Molecules: Cytochromes27:18
- Carrier Molecules: Flavin Mononucleotide (FMN)28:05
- Chemiosmosis32:46
- The Process of Chemiosmosis32:47
- Summary of ATP Produced by Aerobic Respiration38:24
- ATP Produced by Aerobic Respiration38:27
- Example 1: Aerobic Respiration43:38
- Example 2: Label the Location for Each Process and Structure45:08
- Example 3: The Electron Transport Chain47:06
- Example 4: Mitochondrial Inner Membrane48:38
1h 2m 52s
- Intro0:00
- Photosynthesis0:09
- Introduction to Photosynthesis0:10
- Autotrophs and Heterotrophs0:25
- Overview of Photosynthesis Reaction1:05
- Leaf Anatomy and Chloroplast Structure2:54
- Chloroplast2:55
- Cuticle3:16
- Upper Epidermis3:27
- Mesophyll3:40
- Stomates4:00
- Guard Cells4:45
- Transpiration5:01
- Vascular Bundle5:20
- Stroma and Double Membrane6:20
- Grana7:17
- Thylakoids7:30
- Dark Reaction and Light Reaction7:46
- Light Reactions8:43
- Light Reactions8:47
- Pigments: Chlorophyll a, Chlorophyll b, and Carotenoids9:19
- Wave and Particle12:10
- Photon12:34
- Photosystems13:24
- Photosystems13:28
- Reaction-Center Complex and Light Harvesting Complexes14:01
- Noncyclic Photophosphorylation17:46
- Noncyclic Photophosphorylation Overview17:47
- What is Photophosphorylation?18:25
- Noncyclic Photophosphorylation Process19:07
- Photolysis and The Rest of Noncyclic Photophosphorylation21:33
- Cyclic Photophosphorylation31:45
- Cyclic Photophosphorylation31:46
- Light Independent Reactions34:34
- The Calvin Cycle34:35
- C3 Plants and Photorespiration40:31
- C3 Plants and Photorespiration40:32
- C4 Plants45:32
- C4 Plants: Structures and Functions45:33
- CAM Plants50:25
- CAM Plants: Structures and Functions50:35
- Example 1: Calvin Cycle54:34
- Example 2: C4 Plant55:48
- Example 3: Photosynthesis and Photorespiration58:35
- Example 4: CAM Plants1:00:41
38m 45s
- Intro0:00
- Review of DNA Structure0:09
- DNA Molecules0:10
- Nitrogenous Base: Pyrimidines and Purines1:25
- DNA Double Helix3:03
- Complementary Strands of DNA3:12
- 5' to 3' & Antiparallel4:55
- Overview of DNA Replication7:10
- DNA Replication & Semiconservative7:11
- DNA Replication10:26
- Origin of Replication10:28
- Helicase11:10
- Single-Strand Binding Protein12:05
- Topoisomerases13:14
- DNA Polymerase14:26
- Primase15:55
- Leading and Lagging Strands16:51
- Leading Strand and Lagging Strand16:52
- Okazaki Fragments18:10
- DNA Polymerase I20:11
- Ligase21:12
- Proofreading and Mismatch Repair22:18
- Proofreading22:19
- Mismatch23:33
- Telomeres24:58
- Telomeres24:59
- Example 1: Function of Enzymes During DNA Synthesis28:09
- Example 2: Accuracy of the DNA Sequence31:42
- Example 3: Leading Strand and Lagging Strand32:38
- Example 4: Telomeres35:40
1h 17m 1s
- Intro0:00
- Transcription and Translation Overview0:07
- From DNA to RNA to Protein0:09
- Structure and Types of RNA3:14
- Structure and Types of RNA3:33
- mRNA6:19
- rRNA7:02
- tRNA7:28
- Transcription7:54
- Initiation Phase8:11
- Elongation Phase12:12
- Termination Phase14:51
- RNA Processing16:11
- Types of RNA Processing16:12
- Exons and Introns16:35
- Splicing & Spliceosomes18:27
- Addition of a 5' Cap and a Poly A tail20:41
- Alternative Splicing21:43
- Translation23:41
- Nucleotide Triplets or Codons23:42
- Start Codon25:24
- Stop Codons25:38
- Coding of Amino Acids and Wobble Position25:57
- Translation Cont.28:29
- Transfer RNA (tRNA): Structures and Functions28:30
- Ribosomes35:15
- Peptidyl, Aminoacyl, and Exit Site35:23
- Steps of Translation36:58
- Initiation Phase37:12
- Elongation Phase43:12
- Termination Phase45:28
- Mutations49:43
- Types of Mutations49:44
- Substitutions: Silent51:11
- Substitutions: Missense55:27
- Substitutions: Nonsense59:37
- Insertions and Deletions1:01:10
- Example 1: Three Types of Processing that are Performed on pre-mRNA1:06:53
- Example 2: The Process of Translation1:09:10
- Example 3: Transcription1:12:04
- Example 4: Three Types of Substitution Mutations1:14:09
43m 12s
- Intro0:00
- Structure of Viruses0:09
- Structure of Viruses: Capsid and Envelope0:10
- Bacteriophage1:48
- Other Viruses2:28
- Overview of Viral Reproduction3:15
- Host Range3:48
- Step 1: Bind to Host Cell4:39
- Step 2: Viral Nuclei Acids Enter the Cell5:15
- Step 3: Viral Nucleic Acids & Proteins are Synthesized5:54
- Step 4: Virus Assembles6:34
- Step 5: Virus Exits the Cell6:55
- The Lytic Cycle7:37
- Steps in the Lytic Cycle7:38
- The Lysogenic Cycle11:27
- Temperate Phage11:34
- Steps in the Lysogenic Cycle12:09
- RNA Viruses16:57
- Types of RNA Viruses17:15
- Positive Sense18:16
- Negative Sense18:48
- Reproductive Cycle of RNA Viruses19:32
- Retroviruses25:48
- Complementary DNA (cDNA) & Reverse Transcriptase25:49
- Life Cycle of a Retrovirus28:22
- Prions32:42
- Prions: Definition and Examples32:45
- Viroids34:46
- Example 1: The Lytic Cycle35:37
- Example 2: Retrovirus38:03
- Example 3: Positive Sense RNA vs. Negative Sense RNA39:10
- Example 4: The Lysogenic Cycle40:42
49m 45s
- Intro0:00
- Bacterial Genomes0:09
- Structure of Bacterial Genomes0:16
- Transformation1:22
- Transformation1:23
- Vector2:49
- Transduction3:32
- Process of Transduction3:38
- Conjugation8:06
- Conjugation & F factor8:07
- Operons14:02
- Definition and Example of Operon14:52
- Structural Genes16:23
- Promoter Region17:04
- Regulatory Protein & Operators17:53
- The lac Operon20:09
- The lac Operon: Inducible System20:10
- The trp Operon28:02
- The trp Operon: Repressible System28:03
- Corepressor31:37
- Anabolic & Catabolic33:12
- Positive Regulation of the lac Operon34:39
- Positive Regulation of the lac Operon34:40
- Example 1: The Process of Transformation39:07
- Example 2: Operon & Terms43:29
- Example 3: Inducible lac Operon and Repressible trp Operon45:15
- Example 4: lac Operon47:10
54m 26s
- Intro0:00
- Mechanism of Gene Regulation0:11
- Differential Gene Expression0:13
- Levels of Regulation2:24
- Chromatin Structure and Modification4:35
- Chromatin Structure4:36
- Levels of Packing5:50
- Euchromatin and Heterochromatin8:58
- Modification of Chromatin Structure9:58
- Epigenetic12:49
- Regulation of Transcription14:20
- Promoter Region, Exon, and Intron14:26
- Enhancers: Control Element15:31
- Enhancer & DNA-Bending Protein17:25
- Coordinate Control21:23
- Silencers23:01
- Post-Transcriptional Regulation24:05
- Post-Transcriptional Regulation24:07
- Alternative Splicing27:19
- Differences in mRNA Stability28:02
- Non-Coding RNA Molecules: micro RNA & siRNA30:01
- Regulation of Translation and Post-Translational Modifications32:31
- Regulation of Translation and Post-Translational Modifications32:55
- Ubiquitin35:21
- Proteosomes36:04
- Transposons37:50
- Mobile Genetic Elements37:56
- Barbara McClintock38:37
- Transposons & Retrotransposons40:38
- Insertion Sequences43:14
- Complex Transposons43:58
- Example 1: Four Mechanisms that Decrease Production of Protein45:13
- Example 2: Enhancers and Gene Expression49:09
- Example 3: Primary Transcript50:41
- Example 4: Retroviruses and Retrotransposons52:11
49m 26s
- Intro0:00
- Definition of Biotechnology0:08
- Biotechnology0:09
- Genetic Engineering1:05
- Example: Golden Corn1:57
- Recombinant DNA2:41
- Recombinant DNA2:42
- Transformation3:24
- Transduction4:24
- Restriction Enzymes, Restriction Sites, & DNA Ligase5:32
- Gene Cloning13:48
- Plasmids14:20
- Gene Cloning: Step 117:35
- Gene Cloning: Step 217:57
- Gene Cloning: Step 318:53
- Gene Cloning: Step 419:46
- Gel Electrophoresis27:25
- What is Gel Electrophoresis?27:26
- Gel Electrophoresis: Step 128:13
- Gel Electrophoresis: Step 228:24
- Gel Electrophoresis: Step 3 & 428:39
- Gel Electrophoresis: Step 529:55
- Southern Blotting31:25
- Polymerase Chain Reaction (PCR)32:11
- Polymerase Chain Reaction (PCR)32:12
- Denaturing Phase35:40
- Annealing Phase36:07
- Elongation/ Extension Phase37:06
- DNA Sequencing and the Human Genome Project39:19
- DNA Sequencing and the Human Genome Project39:20
- Example 1: Gene Cloning40:40
- Example 2: Recombinant DNA43:04
- Example 3: Match Terms With Descriptions45:43
- Example 4: Polymerase Chain Reaction47:36
1h 32m 8s
- Intro0:00
- Background0:40
- Gregory Mendel & Mendel's Law0:41
- Blending Hypothesis1:04
- Particulate Inheritance2:08
- Terminology2:55
- Gene3:05
- Locus3:57
- Allele4:37
- Dominant Allele5:48
- Recessive Allele7:38
- Genotype9:22
- Phenotype10:01
- Homozygous10:44
- Heterozygous11:39
- Penetrance11:57
- Expressivity14:15
- Mendel's Experiments15:31
- Mendel's Experiments: Pea Plants15:32
- The Law of Segregation21:16
- Mendel's Conclusions21:17
- The Law of Segregation22:57
- Punnett Squares28:27
- Using Punnet Squares28:30
- The Law of Independent Assortment32:35
- Monohybrid32:38
- Dihybrid33:29
- The Law of Independent Assortment34:00
- The Law of Independent Assortment, cont.38:13
- The Law of Independent Assortment: Punnet Squares38:29
- Meiosis and Mendel's Laws43:38
- Meiosis and Mendel's Laws43:39
- Test Crosses49:07
- Test Crosses Example49:08
- Probability: Multiplication Rule and the Addition Rule53:39
- Probability Overview53:40
- Independent Events & Multiplication Rule55:40
- Mutually Exclusive Events & Addition Rule1:00:25
- Incomplete Dominance, Codominance and Multiple Alleles1:02:55
- Incomplete Dominance1:02:56
- Incomplete Dominance, Codominance and Multiple Alleles1:07:06
- Codominance and Multiple Alleles1:07:08
- Polygenic Inheritance and Pleoitropy1:10:19
- Polygenic Inheritance and Pleoitropy1:10:26
- Epistasis1:12:51
- Example of Epistasis1:12:52
- Example 1: Genetic of Eye Color and Height1:17:39
- Example 2: Blood Type1:21:57
- Example 3: Pea Plants1:25:09
- Example 4: Coat Color1:28:34
39m 38s
- Intro0:00
- Review of the Law of Independent Assortment0:14
- Review of the Law of Independent Assortment0:24
- Linked Genes6:06
- Linked Genes6:07
- Bateson & Pannett: Pea Plants8:00
- Crossing Over and Recombination15:17
- Crossing Over and Recombination15:18
- Extranuclear Genes20:50
- Extranuclear Genes20:51
- Cytoplasmic Genes21:31
- Genomic Imprinting23:45
- Genomic Imprinting23:58
- Methylation24:43
- Example 1: Recombination Frequencies & Linkage Map27:07
- Example 2: Linked Genes28:39
- Example 3: Match Terms to Correct Descriptions36:46
- Example 4: Leber's Optic Neuropathy38:40
43m 39s
- Intro0:00
- Sex-Linked Traits0:09
- Human Chromosomes, XY, and XX0:10
- Thomas Morgan's Drosophila1:44
- X-Inactivation and Barr Bodies14:48
- X-Inactivation Overview14:49
- Calico Cats Example17:04
- Pedigrees19:24
- Definition and Example of Pedigree19:25
- Autosomal Dominant Inheritance20:51
- Example: Huntington's Disease20:52
- Autosomal Recessive Inheritance23:04
- Example: Cystic Fibrosis, Tay-Sachs Disease, and Phenylketonuria23:05
- X-Linked Recessive Inheritance27:06
- Example: Hemophilia, Duchene Muscular Dystrohpy, and Color Blindess27:07
- Example 1: Colorblind29:48
- Example 2: Pedigree37:07
- Example 3: Inheritance Pattern39:54
- Example 4: X-inactivation41:17
1h 3m 28s
- Intro0:00
- Background0:09
- Work of Other Scientists0:15
- Aristotle0:43
- Carl Linnaeus1:32
- George Cuvier2:47
- James Hutton4:10
- Thomas Malthus5:05
- Jean-Baptiste Lamark5:45
- Darwin's Theory of Natural Selection7:50
- Evolution8:00
- Natural Selection8:43
- Charles Darwin & The Galapagos Islands10:20
- Genetic Variation20:37
- Mutations20:38
- Independent Assortment21:04
- Crossing Over24:40
- Random Fertilization25:26
- Natural Selection and the Peppered Moth26:37
- Natural Selection and the Peppered Moth26:38
- Types of Natural Selection29:52
- Directional Selection29:55
- Stabilizing Selection32:43
- Disruptive Selection34:21
- Sexual Selection36:18
- Sexual Dimorphism37:30
- Intersexual Selection37:57
- Intrasexual Selection39:20
- Evidence for Evolution40:55
- Paleontology: Fossil Record41:30
- Biogeography45:35
- Continental Drift46:06
- Pangaea46:28
- Marsupials47:11
- Homologous and Analogous Structure50:10
- Homologous Structure50:12
- Analogous Structure53:21
- Example 1: Genetic Variation & Natural Selection56:15
- Example 2: Types of Natural Selection58:07
- Example 3: Mechanisms By Which Genetic Variation is Maintained Within a Population1:00:12
- Example 4: Difference Between Homologous and Analogous Structures1:01:28
53m 22s
- Intro0:00
- Review of Natural Selection0:12
- Review of Natural Selection0:13
- Genetic Drift and Gene Flow4:40
- Definition of Genetic Drift4:41
- Example of Genetic Drift: Cholera Epidemic5:15
- Genetic Drift: Founder Effect7:28
- Genetic Drift: Bottleneck Effect10:27
- Gene Flow13:00
- Quantifying Genetic Variation14:32
- Average Heterozygosity15:08
- Nucleotide Variation17:05
- Maintaining Genetic Variation18:12
- Heterozygote Advantage19:45
- Example of Heterozygote Advantage: Sickle Cell Anemia20:21
- Diploidy23:44
- Geographic Variation26:54
- Frequency Dependent Selection and Outbreeding28:15
- Neutral Traits30:55
- The Hardy-Weinberg Equilibrium31:11
- The Hardy-Weinberg Equilibrium31:49
- The Hardy-Weinberg Conditions32:42
- The Hardy-Weinberg Equation34:05
- The Hardy-Weinberg Example36:33
- Example 1: Match Terms to Descriptions42:28
- Example 2: The Hardy-Weinberg Equilibrium44:31
- Example 3: The Hardy-Weinberg Equilibrium49:10
- Example 4: Maintaining Genetic Variation51:30
51m 2s
- Intro0:00
- Early Life on Earth0:08
- Early Earth0:09
- 1920's Oparin & Haldane0:58
- Abiogenesis2:15
- 1950's Miller & Urey2:45
- Ribozymes5:34
- 3.5 Billion Years Ago6:39
- 2.5 Billion Years Ago7:14
- 1.5 Billion Years Ago7:41
- Endosymbiosis8:00
- 540 Million Years Ago: Cambrian Explosion9:57
- Gradualism and Punctuated Equilibrium11:46
- Gradualism11:47
- Punctuated Equilibrium12:45
- Adaptive Radiation15:08
- Adaptive Radiation15:09
- Example of Adaptive Radiation: Galapogos Islands17:11
- Convergent Evolution, Divergent Evolution, and Coevolution18:30
- Convergent Evolution18:39
- Divergent Evolution21:30
- Coevolution23:49
- Speciation26:27
- Definition and Example of Species26:29
- Reproductive Isolation: Prezygotive27:49
- Reproductive Isolation: Post zygotic29:28
- Allopatric Speciation30:21
- Allopatric Speciation & Geographic Isolation30:28
- Genetic Drift31:31
- Sympatric Speciation34:10
- Sympatric Speciation34:11
- Polyploidy & Autopolyploidy35:12
- Habitat Isolation39:17
- Temporal Isolation41:27
- Selection Selection41:40
- Example 1: Pattern of Evolution42:53
- Example 2: Sympatric Speciation45:16
- Example 3: Patterns of Evolution48:08
- Example 4: Patterns of Evolution49:27
1h 51s
- Intro0:00
- Systems of Classification0:07
- Taxonomy0:08
- Phylogeny1:04
- Phylogenetics Tree1:44
- Cladistics3:37
- Classification of Organisms5:31
- Example of Carl Linnaeus System5:32
- Domains9:26
- Kingdoms: Monera, Protista, Plantae, Fungi, Animalia9:27
- Monera10:06
- Phylogentics Tree: Eurkarya, Bacteria, Archaea11:58
- Domain Eukarya12:50
- Domain Bacteria15:43
- Domain Bacteria15:46
- Pathogens16:41
- Decomposers18:00
- Domain Archaea19:43
- Extremophiles Archaea: Thermophiles and Halophiles19:44
- Methanogens20:58
- Phototrophs, Autotrophs, Chemotrophs and Heterotrophs24:40
- Phototrophs and Chemotrophs25:02
- Autotrophs and Heterotrophs26:54
- Photoautotrophs28:50
- Photoheterotrophs29:28
- Chemoautotrophs30:06
- Chemoheterotrophs31:37
- Domain Eukarya32:40
- Domain Eukarya32:43
- Plant Kingdom34:28
- Protists35:48
- Fungi Kingdom37:06
- Animal Kingdom38:35
- Body Symmetry39:25
- Lack Symetry39:40
- Radial Symmetry: Sea Aneome40:15
- Bilateral Symmetry41:55
- Cephalization43:29
- Germ Layers44:54
- Diploblastic Animals45:18
- Triploblastic Animals45:25
- Ectoderm45:36
- Endoderm46:07
- Mesoderm46:41
- Coelomates47:14
- Coelom47:15
- Acoelomate48:22
- Pseudocoelomate48:59
- Coelomate49:31
- Protosomes50:46
- Deuterosomes51:20
- Example 1: Domains53:01
- Example 2: Match Terms with Descriptions56:00
- Example 3: Kingdom Monera and Domain Archaea57:50
- Example 4: System of Classification59:37
36m 46s
- Intro0:00
- Comparison of Domain Archaea and Domain Bacteria0:08
- Overview of Archaea and Bacteria0:09
- Archaea vs. Bacteria: Nucleus, Organelles, and Organization of Genetic Material1:45
- Archaea vs. Bacteria: Cell Walls2:20
- Archaea vs. Bacteria: Number of Types of RNA Pol2:29
- Archaea vs. Bacteria: Membrane Lipids2:53
- Archaea vs. Bacteria: Introns3:33
- Bacteria: Pathogen4:03
- Bacteria: Decomposers and Fix Nitrogen5:18
- Bacteria: Aerobic, Anaerobic, Strict Anaerobes & Facultative Anaerobes6:02
- Phototrophs, Autotrophs, Heterotrophs and Chemotrophs7:14
- Phototrophs and Chemotrophs7:50
- Autotrophs and Heterotrophs8:53
- Photoautotrophs and Photoheterotrophs10:15
- Chemoautotroph and Chemoheterotrophs11:07
- Structure of Bacteria12:21
- Shapes: Cocci, Bacilli, Vibrio, and Spirochetes12:26
- Structures: Plasma Membrane and Cell Wall14:23
- Structures: Nucleoid Region, Plasmid, and Capsule Basal Apparatus, and Filament15:30
- Structures: Flagella, Basal Apparatus, Hook, and Filament16:36
- Structures: Pili, Fimbrae and Ribosome18:00
- Peptidoglycan: Gram + and Gram -18:50
- Bacterial Genomes and Reproduction21:14
- Bacterial Genomes21:21
- Reproduction of Bacteria22:13
- Transformation23:26
- Vector24:34
- Competent25:15
- Conjugation25:53
- Conjugation: F+ and R Plasmids25:55
- Example 1: Species29:41
- Example 2: Bacteria and Exchange of Genetic Material32:31
- Example 3: Ways in Which Bacteria are Beneficial to Other Organisms33:48
- Example 4: Domain Bacteria vs. Domain Archaea34:53
1h 18m 48s
- Intro0:00
- Classification of Protists0:08
- Classification of Protists0:09
- 'Plant-like' Protists2:06
- 'Animal-like' Protists3:19
- 'Fungus-like' Protists3:57
- Serial Endosymbiosis Theory5:15
- Endosymbiosis Theory5:33
- Photosynthetic Protists7:33
- Life Cycles with a Diploid Adult13:35
- Life Cycles with a Diploid Adult13:56
- Life Cycles with a Haploid Adult15:31
- Life Cycles with a Haploid Adult15:32
- Alternation of Generations17:22
- Alternation of Generations: Multicellular Haploid & Diploid Phase17:23
- Plant-Like Protists19:58
- Euglenids20:43
- Dino Flagellates22:57
- Diatoms26:07
- Plant-Like Protists28:44
- Golden Algae28:45
- Brown Algeas30:05
- Plant-Like Protists33:38
- Red Algae33:39
- Green Algae35:36
- Green Algae: Chlamydomonus37:44
- Animal-Like Protists40:04
- Animal-Like Protists Overview40:05
- Sporozoans (Apicomplexans)40:32
- Alveolates41:41
- Sporozoans (Apicomplexans): Plasmodium & Malaria42:59
- Animal-Like Protists48:44
- Kinetoplastids48:50
- Example of Kinetoplastids: Trypanosomes & African Sleeping Sickness49:30
- Ciliate50:42
- Conjugation53:16
- Conjugation53:26
- Animal-Like Protists57:08
- Parabasilids57:31
- Diplomonads59:06
- Rhizopods1:00:13
- Forams1:02:25
- Radiolarians1:03:28
- Fungus-Like Protists1:04:25
- Fungus-Like Protists Overview1:04:26
- Slime Molds1:05:15
- Cellular Slime Molds: Feeding Stage1:09:21
- Oomycetes1:11:15
- Example 1: Alternation of Generations and Sexual Life Cycles1:13:05
- Example 2: Match Protists to Their Descriptions1:14:12
- Example 3: Three Structures that Protists Use for Motility1:16:22
- Example 4: Paramecium1:17:04
35m 24s
- Intro0:00
- Introduction to Fungi0:09
- Introduction to Fungi0:10
- Mycologist0:34
- Examples of Fungi0:45
- Hyphae, Mycelia, Chitin, and Coencytic Fungi2:26
- Ancestral Protists5:00
- Role of Fungi in the Environment5:35
- Fungi as Decomposers5:36
- Mycorrrhiza6:19
- Lichen8:52
- Life Cycle of Fungi11:32
- Asexual Reproduction11:33
- Sexual Reproduction & Dikaryotic Cell13:16
- Chytridiomycota18:12
- Phylum Chytridiomycota18:17
- Zoospores18:50
- Zygomycota19:07
- Coenocytic & Zygomycota Life Cycle19:08
- Basidiomycota24:27
- Basidiomycota Overview24:28
- Basidiomycota Life Cycle26:11
- Ascomycota28:00
- Ascomycota Overview28:01
- Ascomycota Reproduction28:50
- Example 1: Fungi Fill in the Blank31:02
- Example 2: Name Two Roles Played by Fungi in the Environment32:09
- Example 3: Difference Between Diploid Cell and Dikaryon Cell33:42
- Example 4: Phylum of Fungi, Flagellated Spore, Coencytic34:36
1h 3m 3s
- Intro0:00
- Porifera (Sponges)0:33
- Chordata0:56
- Porifera (Sponges): Sessile, Layers, Aceolomates, and Filter Feeders1:24
- Amoebocytes Cell4:47
- Choanocytes Cell5:56
- Sexual Reproduction6:28
- Cnidaria8:05
- Cnidaria Overview8:06
- Polyp & Medusa: Gastrovasular Cavity8:29
- Cnidocytes9:42
- Anthozoa10:40
- Cubozoa11:23
- Hydrozoa11:53
- Scyphoza13:25
- Platyhelminthes (Flatworms)13:58
- Flatworms: Tribloblastic, Bilateral Symmetry, and Cephalization13:59
- GI System15:33
- Excretory System16:07
- Nervous System17:00
- Turbellarians17:36
- Trematodes18:42
- Monageneans21:32
- Cestoda21:55
- Rotifera (Rotifers)23:45
- Rotifers: Digestive Tract, Pseudocoelem, and Stuctures23:46
- Reproduction: Parthenogenesis25:33
- Nematoda (Roundworms)26:44
- Nematoda (Roundworms)26:45
- Parasites: Pinworms & Hookworms27:26
- Annelida28:36
- Annelida Overview28:37
- Open Circulatory29:21
- Closed Circulatory30:18
- Nervous System31:19
- Excretory System31:43
- Oligochaete32:07
- Leeches33:22
- Polychaetes34:42
- Mollusca35:26
- Mollusca Features35:27
- Major Part 1: Visceral Mass36:21
- Major Part 2: Head-foot Region36:49
- Major Part 3: Mantle37:13
- Radula37:49
- Circulatory, Reproductive, Excretory, and Nervous System38:14
- Major Classes of Molluscs39:12
- Gastropoda39:17
- Polyplacophora40:15
- Bivales40:41
- Cephalopods41:42
- Arthropoda43:35
- Arthropoda Overview43:36
- Segmented Bodies44:14
- Exoskeleton44:52
- Jointed Appendages45:28
- Hemolyph, Excretory & Respiratory System45:41
- Myriapoda & Centipedes47:15
- Cheliceriforms48:20
- Crustcea49:31
- Herapoda50:03
- Echinodermata52:59
- Echinodermata53:00
- Watrer Vascular System54:20
- Selected Characteristics of Invertebrates57:11
- Selected Characteristics of Invertebrates57:12
- Example 1: Phylum Description58:43
- Example 2: Complex Animals59:50
- Example 3: Match Organisms to the Correct Phylum1:01:03
- Example 4: Phylum Arthropoda1:02:01
1h 7s
- Intro0:00
- Phylum Chordata0:06
- Chordates Overview0:07
- Notochord and Dorsal Hollow Nerve Chord1:24
- Pharyngeal Clefts, Arches, and Post-anal Tail3:41
- Invertebrate Chordates6:48
- Lancelets7:13
- Tunicates8:02
- Hagfishes: Craniates8:55
- Vertebrate Chordates10:41
- Veterbrates Overview10:42
- Lampreys11:00
- Gnathostomes12:20
- Six Major Classes of Vertebrates12:53
- chondrichthyes14:23
- Chondrichthyes Overview14:24
- Ectothermic and Endothermic14:42
- Sharks: Lateral Line System, Neuromastsn, and Gills15:27
- Oviparous and Viviparous17:23
- Osteichthyes (Bony Fishes)18:12
- Osteichythes (Bony Fishes) Overview18:13
- Operculum19:05
- Swim Bladder19:53
- Ray-Finned Fishes20:34
- Lobe-Finned Fishes20:58
- Tetrapods22:36
- Tetrapods: Definition and Examples22:37
- Amphibians23:53
- Amphibians Overview23:54
- Order Urodela25:51
- Order Apoda27:03
- Order Anura27:55
- Reptiles30:19
- Reptiles Overview30:20
- Amniotes30:37
- Examples of Reptiles32:46
- Reptiles: Ectotherms, Gas Exchange, and Heart33:40
- Orders of Reptiles34:17
- Sphenodontia, Squamata, Testudines, and Crocodilia34:21
- Birds36:09
- Birds and Dinosaurs36:18
- Theropods38:00
- Birds: High Metabolism, Respiratory System, Lungs, and Heart39:04
- Birds: Endothermic, Bones, and Feathers40:15
- Mammals42:33
- Mammals Overview42:35
- Diaphragm and Heart42:57
- Diphydont43:44
- Synapsids44:41
- Monotremes46:36
- Monotremes46:37
- Marsupials47:12
- Marsupials: Definition and Examples47:16
- Convergent Evolution48:09
- Eutherians (Placental Mammals)49:42
- Placenta49:43
- Order Carnivora50:48
- Order Raodentia51:00
- Order Cetaceans51:14
- Primates51:41
- Primates Overview51:42
- Nails and Hands51:58
- Vision52:51
- Social Care for Young53:28
- Brain53:43
- Example 1: Distinguishing Characteristics of Chordates54:33
- Example 2: Match Description to Correct Term55:56
- Example 3: Bird's Anatomy57:38
- Example 4: Vertebrate Animal, Marine Environment, and Ectothermic59:14
34m 31s
- Intro0:00
- Origin and Classification of Plants0:06
- Origin and Classification of Plants0:07
- Non-Vascular vs. Vascular Plants1:29
- Seedless Vascular & Seed Plants2:28
- Angiosperms & Gymnosperms2:50
- Alternation of Generations3:54
- Alternation of Generations3:55
- Bryophytes7:58
- Overview of Bryrophytes7:59
- Example: Moss Gametophyte9:29
- Example: Moss Sporophyte9:50
- Moss Life Cycle10:12
- Moss Life Cycle10:13
- Seedless Vascular Plants13:23
- Vascular Structures: Cell Walls, and Lignin13:24
- Homosporous17:11
- Heterosporous17:48
- Adaptations to Life on land21:10
- Adaptation 1: Cell Walls21:38
- Adaptation 2: Vascular Plants21:59
- Adaptation 3 : Xylem & Phloem22:31
- Adaptation 4: Seeds23:07
- Adaptation 5: Pollen23:35
- Adaptation 6: Stomata24:45
- Adaptation 7: Reduced Gametophyte Generation25:32
- Example 1: Bryophytes26:39
- Example 2: Sporangium, Lignin, Gametophyte, and Antheridium28:34
- Example 3: Adaptations to Life on Land29:47
- Example 4: Life Cycle of Plant32:06
1h 1m 21s
- Intro0:00
- Plant Tissue0:05
- Dermal Tissue0:15
- Vascular Tissue0:39
- Ground Tissue1:31
- Cell Types in Plants2:14
- Parenchyma Cells2:24
- Collenchyma Cells3:21
- Sclerenchyma Cells3:59
- Xylem5:04
- Xylem: Tracheids and Vessel Elements6:12
- Gymnosperms vs. Angiosperms7:53
- Phloem8:37
- Phloem: Structures and Function8:38
- Sieve-Tube Elements8:45
- Companion Cells & Sieve Plates9:11
- Roots10:08
- Taproots & Fibrous10:09
- Aerial Roots & Prop Roots11:41
- Structures and Functions of Root: Dicot & Monocot13:00
- Pericyle16:57
- The Nitrogen Cylce18:05
- The Nitrogen Cycle18:06
- Mycorrhizae24:20
- Mycorrhizae24:23
- Ectomycorrhiza26:03
- Endomycorrhiza26:25
- Stems26:53
- Stems26:54
- Vascular Bundles of Monocots and Dicots28:18
- Leaves29:48
- Blade & Petiole30:13
- Upper Epidermis, Lower Epidermis & Cuticle30:39
- Ground Tissue, Palisade Mesophyll, Spongy Mesophyll31:35
- Stomata Pores33:23
- Guard Cells34:15
- Vascular Tissues: Vascular Bundles and Bundle Sheath34:46
- Stomata36:12
- Stomata & Gas Exchange36:16
- Guard Cells, Flaccid, and Turgid36:43
- Water Potential38:03
- Factors for Opening Stoma40:35
- Factors Causing Stoma to Close42:44
- Overview of Plant Growth44:23
- Overview of Plant Growth44:24
- Primary Plant Growth46:19
- Apical Meristems46:25
- Root Growth: Zone of Cell Division46:44
- Root Growth: Zone of Cell Elongation47:35
- Root Growth: Zone of Cell Differentiation47:55
- Stem Growth: Leaf Primodia48:16
- Secondary Plant Growth48:48
- Secondary Plant Growth Overview48:59
- Vascular Cambium: Secondary Xylem and Phloem49:38
- Cork Cambium: Periderm and Lenticels51:10
- Example 1: Leaf Structures53:30
- Example 2: List Three Types of Plant Tissue and their Major Functions55:13
- Example 3: What are Two Factors that Stimulate the Opening or Closing of Stomata?56:58
- Example 4: Plant Growth59:18
1h 1m 51s
- Intro0:00
- Seed Plants0:22
- Sporopollenin0:58
- Heterosporous: Megasporangia2:49
- Heterosporous: Microsporangia3:19
- Gymnosperms5:20
- Gymnosperms5:21
- Gymnosperm Life Cycle7:30
- Gymnosperm Life Cycle7:31
- Flower Structure15:15
- Petal & Pollination15:48
- Sepal16:52
- Stamen: Anther, Filament17:05
- Pistill: Stigma, Style, Ovule, Ovary17:55
- Complete Flowers20:14
- Angiosperm Gametophyte Formation20:47
- Male Gametophyte: Microsporocytes, Microsporangia & Meiosis20:57
- Female Gametophyte: Megasporocytes & Meiosis24:22
- Double Fertilization25:43
- Double Fertilization: Pollen Tube and Endosperm25:44
- Angiosperm Life Cycle29:43
- Angiosperm Life Cycle29:48
- Seed Structure and Development33:37
- Seed Structure and Development33:38
- Pollen Dispersal37:53
- Abiotic38:28
- Biotic39:30
- Prevention of Self-Pollination40:48
- Mechanism 141:08
- Mechanism 2: Dioecious41:37
- Mechanism 342:32
- Self-Incompatibility43:08
- Gametophytic Self-Incompatibility44:38
- Sporophytic Self-Incompatibility46:50
- Asexual Reproduction48:33
- Asexual Reproduction & Vegetative Propagation48:34
- Graftiry50:19
- Monocots and Dicots51:34
- Monocots vs.Dicots51:35
- Example 1: Double Fertilization54:43
- Example 2: Mechanisms of Self-Fertilization56:02
- Example 3: Monocots vs. Dicots58:11
- Example 4: Flower Structures1:00:11
40m 30s
- Intro0:00
- Review of Plant Cell Structure0:14
- Cell Wall, Plasma Membrane, Middle lamella, and Cytoplasm0:15
- Plasmodesmata, Chloroplasts, and Central Vacuole3:24
- Water Absorption by Plants4:28
- Root Hairs and Mycorrhizae4:30
- Osmosis and Water Potential5:41
- Apoplast and Symplast Pathways10:01
- Apoplast and Symplast Pathways10:02
- Xylem Structure21:02
- Tracheids and Vessel Elements21:03
- Bulk Flow23:00
- Transpiration23:26
- Cohesion25:10
- Adhesion26:10
- Phloem Structure27:25
- Pholem27:26
- Sieve-Tube Elements27:48
- Companion Cells28:17
- Translocation28:42
- Sugar Source and Sugar Sink Overview28:43
- Example of Sugar Sink30:01
- Example of Sugar Source30:48
- Example 1: Match the Following Terms to their Description33:17
- Example 2: Water Potential34:58
- Example 3: Bulk Flow36:56
- Example 4: Sugar Sink and Sugar Source38:33
48m 10s
- Intro0:00
- Plant Cell Signaling0:17
- Plant Cell Signaling Overview0:18
- Step 1: Reception1:03
- Step 2: Transduction2:32
- Step 3: Response2:58
- Second Messengers3:52
- Protein Kinases4:42
- Auxins6:14
- Auxins6:18
- Indoleacetic Acid (IAA)7:23
- Cytokinins and Gibberellins11:10
- Cytokinins: Apical Dominance & Delay of Aging11:16
- Gibberellins: 'Bolting'13:51
- Ethylene15:33
- Ethylene15:34
- Positive Feedback15:46
- Leaf Abscission18:05
- Mechanical Stress: Triple Response19:36
- Abscisic Acid21:10
- Abscisic Acid21:15
- Tropisms23:11
- Positive Tropism23:50
- Negative Tropism24:07
- Statoliths26:21
- Phytochromes and Photoperiodism27:48
- Phytochromes: PR and PFR27:56
- Circadian Rhythms32:06
- Photoperiod33:13
- Photoperiodism33:38
- Gerner & Allard34:35
- Short-Day Plant35:22
- Long-Day Plant37:00
- Example 1: Plant Hormones41:28
- Example 2: Cytokinins & Gibberellins43:00
- Example 3: Match the Following Terms to their Description44:46
- Example 4: Hormones & Cell Response46:14
48m 14s
- Intro0:00
- Gas Exchange in Animals0:17
- Respiration0:19
- Ventilation1:09
- Characteristics of Respiratory Surfaces1:53
- Gas Exchange in Aquatic Animals3:05
- Simple Aquatic Animals3:06
- Gills & Gas Exchange in Complex Aquatic Animals3:49
- Countercurrent Exchange6:12
- Gas Exchange in Terrestrial Animals13:46
- Earthworms14:07
- Internal Respiratory15:35
- Insects16:55
- Circulatory Fluid19:06
- The Human Respiratory System21:21
- Nasal Cavity, Pharynx, Larynx, and Epiglottis21:50
- Bronchus, Bronchiole, Trachea, and Alveoli23:38
- Pulmonary Surfactants28:05
- Circulatory System: Hemoglobin29:13
- Ventilation30:28
- Inspiration/Expiration: Diaphragm, Thorax, and Abdomen30:33
- Breathing Control Center: Regulation of pH34:34
- Example 1: Tracheal System in Insects39:08
- Example 2: Countercurrent Exchange42:09
- Example 3: Respiratory System44:10
- Example 4: Diaphragm, Ventilation, pH, and Regulation of Breathing45:31
1h 20m 21s
- Intro0:00
- Types of Circulatory Systems0:07
- Circulatory System Overview0:08
- Open Circulatory System3:19
- Closed Circulatory System5:58
- Blood Vessels7:51
- Arteries8:16
- Veins10:01
- Capillaries12:35
- Vasoconstriction and Vasodilation13:10
- Vasoconstriction13:11
- Vasodilation13:47
- Thermoregulation14:32
- Blood15:53
- Plasma15:54
- Cellular Component: Red Blood Cells17:41
- Cellular Component: White Blood Cells20:18
- Platelets21:14
- Blood Types21:35
- Clotting27:04
- Blood, Fibrin, and Clotting27:05
- Hemophilia30:26
- The Heart31:09
- Structures and Functions of the Heart31:19
- Pulmonary and Systemic Circulation40:20
- Double Circuit: Pulmonary Circuit and Systemic Circuit40:21
- The Cardiac Cycle42:35
- The Cardiac Cycle42:36
- Autonomic Nervous System50:00
- Hemoglobin51:25
- Hemoglobin & Hemocyanin51:26
- Oxygen-Hemoglobin Dissociation Curve55:30
- Oxygen-Hemoglobin Dissociation Curve55:44
- Transport of Carbon Dioxide1:06:31
- Transport of Carbon Dioxide1:06:37
- Example 1: Pathway of Blood1:12:48
- Example 2: Oxygenated Blood, Pacemaker, and Clotting1:15:24
- Example 3: Vasodilation and Vasoconstriction1:16:19
- Example 4: Oxygen-Hemoglobin Dissociation Curve1:18:13
56m 11s
- Intro0:00
- Introduction to Digestion0:07
- Digestive Process0:08
- Intracellular Digestion0:45
- Extracellular Digestion1:44
- Types of Digestive Tracts2:08
- Gastrovascular Cavity2:09
- Complete Gastrointestinal Tract (Alimentary Canal)3:54
- 'Crop'4:43
- The Human Digestive System5:41
- Structures of the Human Digestive System5:47
- The Oral Cavity and Esophagus7:47
- Mechanical & Chemical Digestion7:48
- Salivary Glands8:55
- Pharynx and Epigloltis9:43
- Peristalsis11:35
- The Stomach12:57
- Lower Esophageal Sphincter13:00
- Gastric Gland, Parietal Cells, and Pepsin14:32
- Mucus Cell15:48
- Chyme & Pyloric Sphincter17:32
- The Pancreas18:31
- Endocrine and Exocrine19:03
- Amylase20:05
- Proteases20:51
- Lipases22:20
- The Liver23:08
- The Liver & Production of Bile23:09
- The Small Intestine24:37
- The Small Intestine24:38
- Duodenum27:44
- Intestinal Enzymes28:41
- Digestive Enzyme33:30
- Site of Production: Mouth33:43
- Site of Production: Stomach34:03
- Site of Production: Pancreas34:16
- Site of Production: Small Intestine36:18
- Absorption of Nutrients37:51
- Absorption of Nutrients: Jejunum and Ileum37:52
- The Large Intestine44:52
- The Large Intestine: Colon, Cecum, and Rectum44:53
- Regulation of Digestion by Hormones46:55
- Gastrin47:21
- Secretin47:50
- Cholecystokinin (CCK)48:00
- Example 1: Intestinal Cell, Bile, and Digestion of Fats48:29
- Example 2: Matching51:06
- Example 3: Digestion and Absorption of Starch52:18
- Example 4: Large Intestine and Gastric Fluids54:52
1h 12m 14s
- Intro0:00
- Nitrogenous Wastes0:08
- Nitrogenous Wastes Overview0:09
- NH30:39
- Urea2:43
- Uric Acid3:31
- Osmoregulation4:56
- Osmoregulation5:05
- Saltwater Fish vs. Freshwater Fish8:58
- Types of Excretory Systems13:42
- Protonephridia13:50
- Metanephridia16:15
- Malpighian Tubule19:05
- The Human Excretory System20:45
- Kidney, Ureter, bladder, Urethra, Medula, and Cortex20:53
- Filtration, Reabsorption and Secretion22:53
- Filtration22:54
- Reabsorption24:16
- Secretion25:20
- The Nephron26:23
- The Nephron26:24
- The Nephron, cont.41:45
- Descending Loop of Henle41:46
- Ascending Loop of Henle45:45
- Antidiuretic Hormone54:30
- Antidiuretic Hormone (ADH)54:31
- Aldosterone58:58
- Aldosterone58:59
- Example 1: Nephron of an Aquatic Mammal1:04:21
- Example 2: Uric Acid & Saltwater Fish1:06:36
- Example 3: Nephron1:09:14
- Example 4: Gastrointestinal Infection1:10:41
51m 12s
- Intro0:00
- The Endocrine System Overview0:07
- Thyroid0:08
- Exocrine1:56
- Pancreas2:44
- Paracrine Signaling4:06
- Pheromones5:15
- Mechanisms of Hormone Action6:06
- Reception, Transduction, and Response7:06
- Classes of Hormone10:05
- Negative Feedback: Testosterone Example12:16
- The Pancreas15:11
- The Pancreas & islets of Langerhan15:12
- Insulin16:02
- Glucagon17:28
- The Anterior Pituitary19:25
- Thyroid Stimulating Hormone20:24
- Adrenocorticotropic Hormone21:16
- Follide Stimulating Hormone22:04
- Luteinizing Hormone22:45
- Growth Hormone23:45
- Prolactin24:24
- Melanocyte Stimulating Hormone24:55
- The Hypothalamus and Posterior Pituitary25:45
- Hypothalamus, Oxytocin, Antidiuretic Hormone (ADH), and Posterior Pituitary25:46
- The Adrenal Glands31:20
- Adrenal Cortex31:56
- Adrenal Medulla34:29
- The Thyroid35:54
- Thyroxine36:09
- Calcitonin40:27
- The Parathyroids41:44
- Parathyroids Hormone (PTH)41:45
- The Ovaries and Testes43:32
- Estrogen, Progesterone, and Testosterone43:33
- Example 1: Match the Following Hormones with their Descriptions45:38
- Example 2: Pancreas, Endocrine Organ & Exocrine Organ47:06
- Example 3: Insulin and Glucagon48:28
- Example 4: Increased Level of Cortisol in Blood50:25
1h 10m 38s
- Intro0:00
- Types of Nervous Systems0:28
- Nerve Net0:37
- Flatworm1:07
- Cephalization1:52
- Arthropods2:44
- Echinoderms3:11
- Nervous System Organization3:40
- Nervous System Organization Overview3:41
- Automatic Nervous System: Sympathetic & Parasympathetic4:42
- Neuron Structure6:57
- Cell Body & Dendrites7:16
- Axon & Axon Hillock8:20
- Synaptic Terminals, Mylenin, and Nodes of Ranvier9:01
- Pre-synaptic and Post-synaptic Cells10:16
- Pre-synaptic Cells10:17
- Post-synaptic Cells11:05
- Types of Neurons11:50
- Sensory Neurons11:54
- Motor Neurons13:12
- Interneurons14:24
- Resting Potential15:14
- Membrane Potential15:25
- Resting Potential: Chemical Gradient16:06
- Resting Potential: Electrical Gradient19:18
- Gated Ion Channels24:40
- Voltage-Gated & Ligand-Gated Ion Channels24:48
- Action Potential30:09
- Action Potential Overview30:10
- Step 132:07
- Step 232:17
- Step 333:12
- Step 435:14
- Step 536:39
- Action Potential Transmission39:04
- Action Potential Transmission39:05
- Speed of Conduction41:19
- Saltatory Conduction42:58
- The Synapse44:17
- The Synapse: Presynaptic & Postsynaptic Cell44:31
- Examples of Neurotransmitters50:05
- Brain Structure51:57
- Meniges52:19
- Cerebrum52:56
- Corpus Callosum53:13
- Gray & White Matter53:38
- Cerebral Lobes55:35
- Cerebellum56:00
- Brainstem56:30
- Medulla56:51
- Pons57:22
- Midbrain57:55
- Thalamus58:25
- Hypothalamus58:58
- Ventricles59:51
- The Spinal Cord1:00:29
- Sensory Stimuli1:00:30
- Reflex Arc1:01:41
- Example 1: Automatic Nervous System1:04:38
- Example 2: Synaptic Terminal and the Release of Neurotransmitters1:06:22
- Example 3: Volted-Gated Ion Channels1:08:00
- Example 4: Neuron Structure1:09:26
39m 29s
- Intro0:00
- Skeletal System Types and Function0:30
- Skeletal System0:31
- Exoskeleton1:34
- Endoskeleton2:32
- Skeletal System Components2:55
- Bone3:06
- Cartilage5:04
- Tendons6:18
- Ligaments6:34
- Skeletal Muscle6:52
- Skeletal Muscle7:24
- Sarcomere9:50
- The Sliding Filament Theory13:12
- The Sliding Filament Theory: Muscle Contraction13:13
- The Neuromuscular Junction17:24
- The Neuromuscular Junction: Motor Neuron & Muscle Fiber17:26
- Sarcolemma, Sarcoplasmic21:54
- Tropomyosin & Troponin23:35
- Summation and Tetanus25:26
- Single Twitch, Summation of Two Twitches, and Tetanus25:27
- Smooth Muscle28:50
- Smooth Muscle28:58
- Cardiac Muscle30:40
- Cardiac Muscle30:42
- Summary of Muscle Types32:07
- Summary of Muscle Types32:08
- Example 1: Contraction and Skeletal Muscle33:15
- Example 2: Skeletal Muscle and Smooth Muscle36:23
- Example 3: Muscle Contraction, Bone, and Nonvascularized Connective Tissue37:31
- Example 4: Sarcomere38:17
1h 24m 28s
- Intro0:00
- The Lymphatic System0:16
- The Lymphatic System Overview0:17
- Function 11:23
- Function 22:27
- Barrier Defenses3:41
- Nonspecific vs. Specific Immune Defenses3:42
- Barrier Defenses5:12
- Nonspecific Cellular Defenses7:50
- Nonspecific Cellular Defenses Overview7:53
- Phagocytes9:29
- Neutrophils11:43
- Macrophages12:15
- Natural Killer Cells12:55
- Inflammatory Response14:19
- Complement18:16
- Interferons18:40
- Specific Defenses - Acquired Immunity20:12
- T lymphocytes and B lymphocytes20:13
- B Cells23:35
- B Cells & Humoral Immunity23:41
- Clonal Selection29:50
- Clonal Selection29:51
- Primary Immune Response34:28
- Secondary Immune Response35:31
- Cytotoxic T Cells38:41
- Helper T Cells39:20
- Major Histocompatibility Complex Molecules40:44
- Major Histocompatibility Complex Molecules40:55
- Helper T Cells52:36
- Helper T Cells52:37
- Mechanisms of Antibody Action59:00
- Mechanisms of Antibody Action59:01
- Opsonization1:00:01
- Complement System1:01:57
- Classes of Antibodies1:02:45
- IgM1:03:01
- IgA1:03:17
- IgG1:03:53
- IgE1:04:10
- Passive and Active Immunity1:05:00
- Passive Immunity1:05:01
- Active Immunity1:07:49
- Recognition of Self and Non-Self1:09:32
- Recognition of Self and Non-Self1:09:33
- Self-Tolerance & Autoimmune Diseases1:10:50
- Immunodeficiency1:13:27
- Immunodeficiency1:13:28
- Chemotherapy1:13:56
- AID1:14:27
- Example 1: Match the Following Terms with their Descriptions1:15:26
- Example 2: Three Components of Non-specific Immunity1:17:59
- Example 3: Immunodeficient1:21:19
- Example 4: Self-tolerance and Autoimmune Diseases1:23:07
1h 1m 41s
- Intro0:00
- Asexual Reproduction0:17
- Fragmentation0:53
- Fission1:54
- Parthenogenesis2:38
- Sexual Reproduction4:00
- Sexual Reproduction4:01
- Hermaphrodite8:08
- The Male Reproduction System8:54
- Seminiferous Tubules & Leydig Cells8:55
- Epididymis9:48
- Seminal Vesicle11:19
- Bulbourethral12:37
- The Female Reproductive System13:25
- Ovaries13:28
- Fallopian14:50
- Endometrium, Uterus, Cilia, and Cervix15:03
- Mammary Glands16:44
- Spermatogenesis17:08
- Spermatogenesis17:09
- Oogenesis21:01
- Oogenesis21:02
- The Menstrual Cycle27:56
- The Menstrual Cycle: Ovarian and Uterine Cycle27:57
- Summary of the Ovarian and Uterine Cycles42:54
- Ovarian42:55
- Uterine44:51
- Oxytocin and Prolactin46:33
- Oxytocin46:34
- Prolactin47:00
- Regulation of the Male Reproductive System47:28
- Hormones: GnRH, LH, FSH, and Testosterone47:29
- Fertilization50:11
- Fertilization50:12
- Structures of Egg50:28
- Acrosomal Reaction51:36
- Cortical Reaction53:09
- Example 1: List Three Differences between Spermatogenesis and oogenesis55:36
- Example 2: Match the Following Terms to their Descriptions57:34
- Example 3: Pregnancy and the Ovarian Cycle58:44
- Example 4: Hormone1:00:43
50m 5s
- Intro0:00
- Cleavage0:31
- Cleavage0:32
- Meroblastic2:06
- Holoblastic Cleavage3:23
- Protostomes4:34
- Deuterostomes5:13
- Totipotent5:52
- Blastula Formation6:42
- Blastula6:46
- Gastrula Formation8:12
- Deuterostomes11:02
- Protostome11:44
- Ectoderm12:17
- Mesoderm12:55
- Endoderm13:40
- Cytoplasmic Determinants15:19
- Cytoplasmic Determinants15:23
- The Bird Embryo22:52
- Cleavage23:35
- Blastoderm23:55
- Primitive Streak25:38
- Migration and Differentiation27:09
- Extraembryonic Membranes28:33
- Extraembryonic Membranes28:34
- Chorion30:02
- Yolk Sac30:36
- Allantois31:04
- The Mammalian Embryo32:18
- Cleavage32:28
- Blastocyst32:44
- Trophoblast34:37
- Following Implantation35:48
- Organogenesis37:04
- Organogenesis, Notochord and Neural Tube37:05
- Induction40:15
- Induction40:39
- Fate Mapping41:40
- Example 1: Processes and Stages of Embryological Development42:49
- Example 2: Transplanted Cells44:33
- Example 3: Germ Layer46:41
- Example 4: Extraembryonic Membranes47:28
47m 48s
- Intro0:00
- Introduction to Animal Behavior0:05
- Introduction to Animal Behavior0:06
- Ethology1:04
- Proximate Cause & Ultimate Cause1:46
- Fixed Action Pattern3:07
- Sign Stimulus3:40
- Releases and Example3:55
- Exploitation and Example7:23
- Learning8:56
- Habituation, Associative Learning, and Imprinting8:57
- Habituation10:03
- Habituation: Definition and Example10:04
- Associative Learning11:47
- Classical12:19
- Operant Conditioning13:40
- Positive & Negative Reinforcement14:59
- Positive & Negative Punishment16:13
- Extinction17:28
- Imprinting17:47
- Imprinting: Definition and Example17:48
- Social Behavior20:12
- Cooperation20:38
- Agonistic21:37
- Dorminance Heirarchies23:23
- Territoriality24:08
- Altruism24:55
- Communication26:56
- Communication26:57
- Mating32:38
- Mating Overview32:40
- Promiscuous33:13
- Monogamous33:32
- Polygamous33:48
- Intrasexual34:22
- Intersexual Selection35:08
- Foraging36:08
- Optimal Foraging Model36:39
- Foraging37:47
- Movement39:12
- Kinesis39:20
- Taxis40:17
- Migration40:54
- Lunar Cycles42:02
- Lunar Cycles42:08
- Example 1: Types of Conditioning43:19
- Example 2: Match the Following Terms to their Descriptions44:12
- Example 3: How is the Optimal Foraging Model Used to Explain Foraging Behavior45:47
- Example 4: Learning46:54
58m 49s
- Intro0:00
- Ecology0:08
- Ecology0:14
- Environment0:22
- Integrates1:41
- Environment Impacts2:20
- Population and Distribution3:20
- Population3:21
- Range4:50
- Potential Range5:10
- Abiotic5:46
- Biotic6:22
- Climate7:55
- Temperature8:40
- Precipitation10:00
- Wind10:37
- Sunlight10:54
- Macroclimates & Microclimates11:31
- Other Abiotic Factors12:20
- Geography12:28
- Water13:17
- Soil and Rocks13:48
- Sunlight14:42
- Sunlight14:43
- Seasons15:43
- June Solstice, December Solstice, March Equinox, and September Equinox15:44
- Tropics19:00
- Seasonability19:39
- Wind and Weather Patterns20:44
- Vertical Circulation20:51
- Surface Wind Patterns25:18
- Local Climate Effects26:51
- Local Climate Effects26:52
- Terrestrial Biomes30:04
- Biome30:05
- Forest31:02
- Tropical Forest32:00
- Tropical Forest32:01
- Temperate Broadleaf Forest32:55
- Temperate Broadleaf Forest32:56
- Coniferous/Taiga Forest34:10
- Coniferous/Taiga Forest34:11
- Desert36:05
- Desert36:06
- Grassland37:45
- Grassland37:46
- Tundra40:09
- Tundra40:10
- Freshwater Biomes42:25
- Freshwater Biomes: Zones42:27
- Eutrophic Lakes44:24
- Oligotrophic Lakes45:01
- Lakes Turnover46:03
- Rivers46:51
- Wetlands47:40
- Estuary48:11
- Marine Biomes48:45
- Marine Biomes: Zones48:46
- Example 1: Diversity of Life52:18
- Example 2: Marine Biome53:08
- Example 3: Season54:20
- Example 4: Biotic vs. Abiotic55:54
41m 16s
- Intro0:00
- Population0:07
- Size 'N'0:16
- Density0:41
- Dispersion1:01
- Measure Population: Count Individuals, Sampling, and Proxymeasure2:26
- Mortality7:29
- Mortality and Survivorship7:30
- Age Structure Diagrams11:52
- Expanding with Rapid Growth, Expanding, and Stable11:58
- Population Growth15:39
- Biotic Potential & Exponential Growth15:43
- Logistic Population Growth19:07
- Carrying Capacity (K)19:18
- Limiting Factors20:55
- Logistic Model and Oscillation22:55
- Logistic Model and Oscillation22:56
- Changes to the Carrying Capacity24:36
- Changes to the Carrying Capacity24:37
- Growth Strategies26:07
- 'r-selected' or 'r-strategist'26:23
- 'K-selected' or 'K-strategist'27:47
- Human Population30:15
- Human Population and Exponential Growth30:21
- Case Study - Lynx and Hare31:54
- Case Study - Lynx and Hare31:55
- Example 1: Estimating Population Size34:35
- Example 2: Population Growth36:45
- Example 3: Carrying Capacity38:17
- Example 4: Types of Dispersion40:15
1h 6m 26s
- Intro0:00
- Community0:07
- Ecosystem0:40
- Interspecific Interactions1:14
- Competition2:45
- Competition Overview2:46
- Competitive Exclusion3:57
- Resource Partitioning4:45
- Character Displacement6:22
- Predation7:46
- Predation7:47
- True Predation8:05
- Grazing/ Herbivory8:39
- Predator Adaptation10:13
- Predator Strategies10:22
- Physical Features11:02
- Prey Adaptation12:14
- Prey Adaptation12:23
- Aposematic Coloration13:35
- Batesian Mimicry14:32
- Size15:42
- Parasitism16:48
- Symbiotic Relationship16:54
- Ectoparasites18:31
- Endoparasites18:53
- Hyperparisitism19:21
- Vector20:08
- Parasitoids20:54
- Mutualism21:23
- Resource - Resource mutualism21:34
- Service - Resource Mutualism23:31
- Service - Service Mutualism: Obligate & Facultative24:23
- Commensalism26:01
- Commensalism26:03
- Symbiosis27:31
- Trophic Structure28:35
- Producers & Consumers: Autotrophs & Heterotrophs28:36
- Food Chain33:26
- Producer & Consumers33:38
- Food Web39:01
- Food Web39:06
- Significant Species within Communities41:42
- Dominant Species41:50
- Keystone Species42:44
- Foundation Species43:41
- Community Dynamics and Disturbances44:31
- Disturbances44:33
- Duration47:01
- Areal Coverage47:22
- Frequency47:48
- Intensity48:04
- Intermediate Level of Disturbance48:20
- Ecological Succession50:29
- Primary and Secondary Ecological Succession50:30
- Example 1: Competition Situation & Outcome57:18
- Example 2: Food Chains1:00:08
- Example 3: Ecological Units1:02:44
- Example 4: Disturbances & Returning to the Original Climax Community1:04:30
57m 42s
- Intro0:00
- Ecosystem: Biotic & Abiotic Components0:15
- First Law of Thermodynamics & Energy Flow0:40
- Gross Primary Productivity (GPP)3:52
- Net Primary Productivity (NPP)4:50
- Biogeochemical Cycles7:16
- Law of Conservation of Mass & Biogeochemical Cycles7:17
- Water Cycle10:55
- Water Cycle10:57
- Carbon Cycle17:52
- Carbon Cycle17:53
- Nitrogen Cycle22:40
- Nitrogen Cycle22:41
- Phosphorous Cycle29:34
- Phosphorous Cycle29:35
- Climate Change33:20
- Climate Change33:21
- Eutrophication39:38
- Nitrogen40:34
- Phosphorous41:29
- Eutrophication42:55
- Example 1: Energy and Ecosystems45:28
- Example 2: Atmospheric CO248:44
- Example 3: Nitrogen Cycle51:22
- Example 4: Conversion of a Forest near a Lake to Farmland53:20
2h 4m 30s
- Intro0:00
- Lab 1: Diffusion and Osmosis0:09
- Lab 1: Diffusion and Osmosis0:10
- Lab 1: Water Potential11:55
- Lab 1: Water Potential11:56
- Lab 2: Enzyme Catalysis18:30
- Lab 2: Enzyme Catalysis18:31
- Lab 3: Mitosis and Meiosis27:40
- Lab 3: Mitosis and Meiosis27:41
- Lab 3: Mitosis and Meiosis31:50
- Ascomycota Life Cycle31:51
- Lab 4: Plant Pigments and Photosynthesis40:36
- Lab 4: Plant Pigments and Photosynthesis40:37
- Lab 5: Cell Respiration49:56
- Lab 5: Cell Respiration49:57
- Lab 6: Molecular Biology55:06
- Lab 6: Molecular Biology & Transformation 1st Part55:07
- Lab 6: Molecular Biology1:01:16
- Lab 6: Molecular Biology 2nd Part1:01:17
- Lab 7: Genetics of Organisms1:07:32
- Lab 7: Genetics of Organisms1:07:33
- Lab 7: Chi-square Analysis1:13:00
- Lab 7: Chi-square Analysis1:13:03
- Lab 8: Population Genetics and Evolution1:20:41
- Lab 8: Population Genetics and Evolution1:20:42
- Lab 9: Transpiration1:24:02
- Lab 9: Transpiration1:24:03
- Lab 10: Physiology of the Circulatory System1:31:05
- Lab 10: Physiology of the Circulatory System1:31:06
- Lab 10: Temperature and Metabolism in Ectotherms1:38:25
- Lab 10: Temperature and Metabolism in Ectotherms1:38:30
- Lab 11: Animal Behavior1:40:52
- Lab 11: Animal Behavior1:40:53
- Lab 12: Dissolved Oxygen & Aquatic Primary Productivity1:45:36
- Lab 12: Dissolved Oxygen & Aquatic Primary Productivity1:45:37
- Lab 12: Primary Productivity1:49:06
- Lab 12: Primary Productivity1:49:07
- Example 1: Chi-square Analysis1:56:31
- Example 2: Mitosis1:59:28
- Example 3: Transpiration of Plants2:00:27
- Example 4: Population Genetic2:01:16
13m 2s
- Intro0:00
- AP Biology Structure0:18
- Section I0:31
- Section II1:16
- Scoring2:04
- The Four 'Big Ideas'3:51
- Process of Evolution4:37
- Biological Systems Utilize4:44
- Living Systems4:55
- Biological Systems Interact5:03
- Items to Bring to the Test7:56
- Test Taking Tips9:53
1h 4m 29s
- Intro0:00
- AP Biology Practice Exam0:14
- Multiple Choice 10:40
- Multiple Choice 22:27
- Multiple Choice 34:30
- Multiple Choice 46:43
- Multiple Choice 59:27
- Multiple Choice 611:32
- Multiple Choice 712:54
- Multiple Choice 814:42
- Multiple Choice 917:06
- Multiple Choice 1018:42
- Multiple Choice 1120:49
- Multiple Choice 1223:23
- Multiple Choice 1326:20
- Multiple Choice 1427:52
- Multiple Choice 1528:44
- Multiple Choice 1633:07
- Multiple Choice 1735:31
- Multiple Choice 1839:43
- Multiple Choice 1940:37
- Multiple Choice 2042:47
- Multiple Choice 2145:58
- Multiple Choice 2249:49
- Multiple Choice 2353:44
- Multiple Choice 2455:12
- Multiple Choice 2555:59
- Multiple Choice 2656:50
- Multiple Choice 2758:08
- Multiple Choice 2859:54
- Multiple Choice 291:01:36
- Multiple Choice 301:02:31
- Multiple Choice 311:03:50
50m 44s
- Intro0:00
- AP Biology Practice Exam0:14
- Multiple Choice 320:27
- Multiple Choice 334:14
- Multiple Choice 345:12
- Multiple Choice 356:51
- Multiple Choice 3610:46
- Multiple Choice 3711:27
- Multiple Choice 3812:17
- Multiple Choice 3913:49
- Multiple Choice 4017:02
- Multiple Choice 4118:27
- Multiple Choice 4219:35
- Multiple Choice 4321:10
- Multiple Choice 4423:35
- Multiple Choice 4525:00
- Multiple Choice 4626:20
- Multiple Choice 4728:40
- Multiple Choice 4830:14
- Multiple Choice 4931:24
- Multiple Choice 5032:45
- Multiple Choice 5133:41
- Multiple Choice 5234:40
- Multiple Choice 5336:12
- Multiple Choice 5438:06
- Multiple Choice 5538:37
- Multiple Choice 5640:00
- Multiple Choice 5741:18
- Multiple Choice 5843:12
- Multiple Choice 5944:25
- Multiple Choice 6045:02
- Multiple Choice 6146:10
- Multiple Choice 6247:54
- Multiple Choice 6349:01
21m 52s
- Intro0:00
- AP Biology Practice Exam0:17
- Grid In Question 10:29
- Grid In Question 23:49
- Grid In Question 311:04
- Grid In Question 413:18
- Grid In Question 517:01
- Grid In Question 619:30
31m 22s
- Intro0:00
- AP Biology Practice Exam0:18
- Free Response 10:29
- Free Response 220:47
24m 41s
- Intro0:00
- AP Biology Practice Exam0:15
- Free Response 30:26
- Free Response 45:21
- Free Response 58:25
- Free Response 611:38
- Free Response 714:48
- Free Response 822:14
For more information, please see full course syllabus of AP Biology
AP Biology Mendelian Genetics
Gregory Mendel introduced the idea of dominant and recessive traits and particulate inheritance by experimenting on pea plants. Alleles are options for the expression of a gene. The gene for eye color has multiple alleles: some for blue eyes and some for brown eyes. Recessive alleles, like those for blue eyes, require two copies to be expressed. Punnett Squares show all the possible combinations of dominant and recessive genes according to the law of independent assortment and probability. Polygenic traits are controlled by more than one gene. Pleiotropic traits are sets of multiple traits that can be controlled by one gene.
Share this knowledge with your friends!
Copy & Paste this embed code into your website’s HTML
Please ensure that your website editor is in text mode when you paste the code.(In Wordpress, the mode button is on the top right corner.)
- - Allow users to view the embedded video in full-size.
0 answers
Post by Hammal on October 30, 2021
Hello Professor ! can you give another example of co-dominance ?
2 answers
Last reply by: Andrew Cheesman
Thu Feb 12, 2015 10:44 AM
Post by martin yu on January 19, 2014
The last question about the albino with the punnett square.
Cc x Cc - shouldn't cc = 1/4; why 1/2?
1 answer
Wed Mar 26, 2014 6:34 PM
Post by Muhammad Ziad on January 11, 2014
Hi Dr. Eaton, I am still a little confused on epistasis. Could you clarify it a little bit?
1 answer
Wed Nov 6, 2013 1:18 AM
Post by Maddie G on October 31, 2013
How do alleles differ from variations of genes or single nucleotide polymorphisms?
Particularly in terms of addictions- if a study says they investigated single nucleotide polymorphisms, and that a variant of gene increases vulnerability, how are these two linked? Or are they basically the same thing
0 answers
Post by Omar Younes on October 8, 2013
Hello,
are we not able to fast forward videos?
1 answer
Sun Jan 19, 2014 4:02 PM
Post by bo young lee on March 5, 2013
where can i find more genetic video in your section? (i really like your teaching style then other teacher in educator.com)
1 answer
Wed Jan 9, 2013 2:16 AM
Post by Ramitha Manivannan on December 22, 2012
Dr. Eaton, can I please have your email address so I can ask you questions on this lecture?
1 answer
Fri Dec 9, 2011 12:59 PM
Post by Cameron Saghaiepour on December 8, 2011
I still don't understand law of independent assortment.
1 answer
Fri Dec 9, 2011 12:54 PM
Post by Chris Hahn on December 6, 2011
Example 1:
What is the probability that the child will be tall and have brown eyes?
I thought it was (1/16) x (9/16) = 9/256
0 answers
Post by Sai Nettyam on November 6, 2011
Dr. Easton,
Thank you for the great videos.
0 answers
Post by Billy Jay on April 13, 2011
Little error towards the end. The Test Cross should consist of: CC, Cc, cC, and cc with a 1/4 probability.
1 answer
Last reply by: Ramin Sadat
Thu Sep 5, 2013 7:46 PM
Post by Billy Jay on April 13, 2011
Hi Dr. Eaton,
Do you think the information organized into these series of lectures (on Genetics) are sufficient enough for the Genetics portion of MCAT?