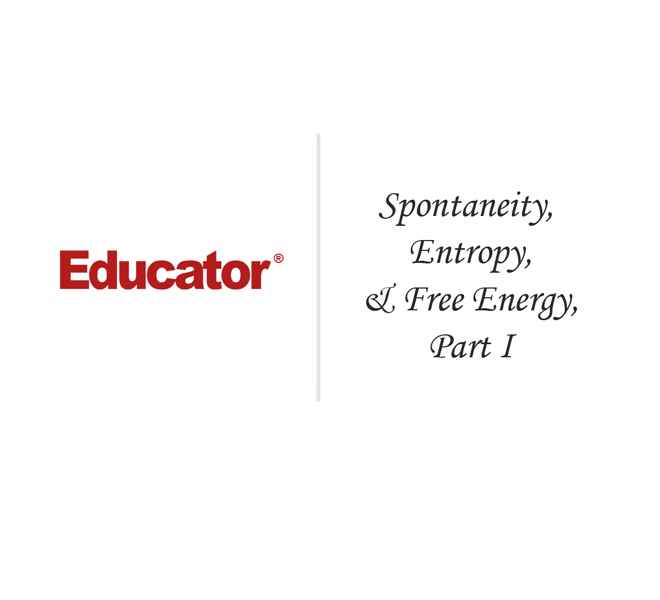
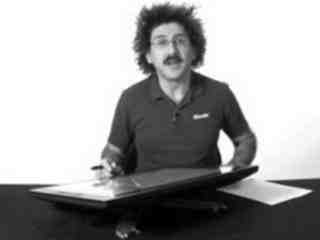
Raffi Hovasapian
Spontaneity, Entropy, & Free Energy, Part I
Slide Duration:Table of Contents
41m 24s
- Intro0:00
- Periodic Table of Elements0:15
- Naming Compounds3:13
- Definition and Examples of Ions3:14
- Ionic (Symbol to Name): NaCl5:23
- Ionic (Name to Symbol): Calcium Oxide7:58
- Ionic - Polyatoms Anions: Examples12:45
- Ionic - Polyatoms Anions (Symbol to Name): KClO14:50
- Ionic - Polyatoms Anions (Name to Symbol): Potassium Phosphate15:49
- Ionic Compounds Involving Transition Metals (Symbol to Name): Co₂(CO₃)₃20:48
- Ionic Compounds Involving Transition Metals (Name to Symbol): Palladium 2 Acetate22:44
- Naming Covalent Compounds (Symbol to Name): CO26:21
- Naming Covalent Compounds (Name to Symbol): Nitrogen Trifluoride27:34
- Naming Covalent Compounds (Name to Symbol): Dichlorine Monoxide27:57
- Naming Acids Introduction28:11
- Naming Acids (Name to Symbol): Chlorous Acid35:08
- % Composition by Mass Example37:38
37m 19s
- Intro0:00
- Stoichiometry0:25
- Introduction to Stoichiometry0:26
- Example 15:03
- Example 210:17
- Example 315:09
- Example 424:02
- Example 5: Questions28:11
- Example 5: Part A - Limiting Reactant30:30
- Example 5: Part B32:27
- Example 5: Part C35:00
31m 14s
- Intro0:00
- Precipitation Reactions0:53
- Dissociation of ionic Compounds0:54
- Solubility Guidelines for ionic Compounds: Soluble Ionic Compounds8:15
- Solubility Guidelines for ionic Compounds: Insoluble ionic Compounds12:56
- Precipitation Reactions14:08
- Example 1: Mixing a Solution of BaCl₂ & K₂SO₄21:21
- Example 2: Mixing a Solution of Mg(NO₃)₂ & KI26:10
43m 21s
- Intro0:00
- Acid-Base Reactions1:00
- Introduction to Acid: Monoprotic Acid and Polyprotic Acid1:01
- Introduction to Base8:28
- Neutralization11:45
- Example 116:17
- Example 221:55
- Molarity24:50
- Example 326:50
- Example 430:01
- Example 4: Limiting Reactant37:51
- Example 4: Reaction Part40:01
47m 58s
- Intro0:00
- Oxidation Reduction Reactions0:26
- Oxidation and Reduction Overview0:27
- How Can One Tell Whether Oxidation-Reduction has Taken Place?7:13
- Rules for Assigning Oxidation State: Number 111:22
- Rules for Assigning Oxidation State: Number 212:46
- Rules for Assigning Oxidation State: Number 313:25
- Rules for Assigning Oxidation State: Number 414:50
- Rules for Assigning Oxidation State: Number 515:41
- Rules for Assigning Oxidation State: Number 617:00
- Example 1: Determine the Oxidation State of Sulfur in the Following Compounds18:20
- Activity Series and Reduction Properties25:32
- Activity Series and Reduction Properties25:33
- Example 2: Write the Balance Molecular, Total Ionic, and Net Ionic Equations for Al + HCl31:37
- Example 334:25
- Example 437:55
31m 50s
- Intro0:00
- Stoichiometry Example 10:36
- Example 1: Question and Answer0:37
- Stoichiometry Example 26:57
- Example 2: Questions6:58
- Example 2: Part A Solution12:16
- Example 2: Part B Solution13:05
- Example 2: Part C Solution14:00
- Example 2: Part D Solution14:38
- Stoichiometry Example 317:56
- Example 3: Questions17:57
- Example 3: Part A Solution19:51
- Example 3: Part B Solution21:43
- Example 3: Part C Solution26:46
49m 40s
- Intro0:00
- Pressure0:22
- Pressure Overview0:23
- Torricelli: Barometer4:35
- Measuring Gas Pressure in a Container7:49
- Boyle's Law12:40
- Example 116:56
- Gas Laws21:18
- Gas Laws21:19
- Avogadro's Law26:16
- Example 231:47
- Ideal Gas Equation38:20
- Standard Temperature and Pressure (STP)38:21
- Example 340:43
32m
- Intro0:00
- Gases0:27
- Gases0:28
- Mole Fractions5:52
- Vapor Pressure8:22
- Example 113:25
- Example 222:45
31m 58s
- Intro0:00
- Kinetic Molecular Theory and Real Gases0:45
- Kinetic Molecular Theory 10:46
- Kinetic Molecular Theory 24:23
- Kinetic Molecular Theory 35:42
- Kinetic Molecular Theory 46:27
- Equations7:52
- Effusion11:15
- Diffusion13:30
- Example 119:54
- Example 223:23
- Example 326:45
25m 34s
- Intro0:00
- Example 10:34
- Example 10:35
- Example 26:15
- Example 2: Part A6:16
- Example 2: Part B8:46
- Example 2: Part C10:30
- Example 2: Part D11:15
- Example 2: Part E12:20
- Example 2: Part F13:22
- Example 314:45
- Example 314:46
- Example 418:16
- Example 418:17
- Example 521:04
- Example 521:05
37m 32s
- Intro0:00
- Thermochemistry0:25
- Temperature and Heat0:26
- Work3:07
- System, Surroundings, Exothermic Process, and Endothermic Process8:19
- Work & Gas: Expansion and Compression16:30
- Example 124:41
- Example 227:47
- Example 331:58
32m 34s
- Intro0:00
- Thermochemistry1:43
- Defining Enthalpy & Hess's Law1:44
- Example 16:48
- State Function13:11
- Example 217:15
- Example 324:09
23m 9s
- Intro0:00
- Thermochemistry1:04
- Standard Enthalpy of Formation: Definition & Equation1:05
- ∆H of Formation10:00
- Example 111:22
- Example 219:00
39m 28s
- Intro0:00
- Thermochemistry0:21
- Heat Capacity0:22
- Molar Heat Capacity4:44
- Constant Pressure Calorimetry5:50
- Example 112:24
- Constant Volume Calorimetry21:54
- Example 224:40
- Example 331:03
36m 24s
- Intro0:00
- Kinetics2:18
- Rate: 2 NO₂ (g) → 2NO (g) + O₂ (g)2:19
- Reaction Rates Graph7:25
- Time Interval & Average Rate13:13
- Instantaneous Rate15:13
- Rate of Reaction is Proportional to Some Power of the Reactant Concentrations23:49
- Example 127:19
30m 48s
- Intro0:00
- Kinetics0:33
- Rate0:34
- Idea2:24
- Example 1: NH₄⁺ + NO₂⁻ → NO₂ (g) + 2 H₂O5:36
- Example 2: BrO₃⁻ + 5 Br⁻ + 6 H⁺ → 3 Br₂ + 3 H₂O19:29
32m 17s
- Intro0:00
- Kinetics0:52
- Integrated Rate Law0:53
- Example 16:26
- Example 215:19
- Half-life of a Reaction20:40
- Example 3: Part A25:41
- Example 3: Part B28:01
26m 40s
- Intro0:00
- Kinetics0:22
- Second Order0:23
- Example 16:08
- Zero-Order16:36
- Summary for the Kinetics Associated with the Reaction21:27
40m 59s
- Intro0:00
- Kinetics0:53
- Rate Constant0:54
- Collision Model2:45
- Activation Energy5:11
- Arrhenius Proposed9:54
- 2 Requirements for a Successful Reaction15:39
- Rate Constant17:53
- Arrhenius Equation19:51
- Example 125:00
- Activation Energy & the Values of K32:12
- Example 236:46
29m 8s
- Intro0:00
- Kinetics0:43
- Example 10:44
- Example 26:53
- Example 38:58
- Example 411:36
- Example 516:36
- Example 6: Part A21:00
- Example 6: Part B25:09
46m
- Intro0:00
- Equilibrium1:32
- Introduction to Equilibrium1:33
- Equilibrium Rules14:00
- Example 1: Part A16:46
- Example 1: Part B18:48
- Example 1: Part C22:13
- Example 1: Part D24:55
- Example 2: Part A27:46
- Example 2: Part B31:22
- Example 2: Part C33:00
- Reverse a Reaction36:04
- Example 337:24
40m 53s
- Intro0:00
- Equilibrium1:31
- Equilibriums Involving Gases1:32
- General Equation10:11
- Example 1: Question11:55
- Example 1: Answer13:43
- Example 2: Question19:08
- Example 2: Answer21:37
- Example 3: Question33:40
- Example 3: Answer35:24
45m 53s
- Intro0:00
- Equilibrium0:57
- Reaction Quotient0:58
- If Q > K5:37
- If Q < K6:52
- If Q = K7:45
- Example 1: Part A8:24
- Example 1: Part B13:11
- Example 2: Question20:04
- Example 2: Answer22:15
- Example 3: Question30:54
- Example 3: Answer32:52
- Steps in Solving Equilibrium Problems42:40
31m 51s
- Intro0:00
- Equilibrium1:09
- Example 1: Question1:10
- Example 1: Answer4:15
- Example 2: Question13:04
- Example 2: Answer15:20
- Example 3: Question25:03
- Example 3: Answer26:32
40m 52s
- Intro0:00
- Le Chatelier1:05
- Le Chatelier Principle1:06
- Concentration: Add 'x'5:25
- Concentration: Subtract 'x'7:50
- Example 19:44
- Change in Pressure12:53
- Example 220:40
- Temperature: Exothermic and Endothermic24:33
- Example 329:55
- Example 435:30
50m 11s
- Intro0:00
- Acids and Bases1:14
- Bronsted-Lowry Acid-Base Model1:28
- Reaction of an Acid with Water4:36
- Acid Dissociation10:51
- Acid Strength13:48
- Example 121:22
- Water as an Acid & a Base25:25
- Example 2: Part A32:30
- Example 2: Part B34:47
- Example 3: Part A35:58
- Example 3: Part B39:33
- pH Scale41:12
- Example 443:56
43m 52s
- Intro0:00
- pH of Weak Acid Solutions1:12
- pH of Weak Acid Solutions1:13
- Example 16:26
- Example 214:25
- Example 324:23
- Example 430:38
43m 4s
- Intro0:00
- Bases0:33
- Percent Dissociation: Strong & Weak Bases0:45
- Example 16:23
- Strong Base Dissociation11:24
- Example 213:02
- Weak Acid and General Reaction17:38
- Example: NaOH → Na⁺ + OH⁻20:30
- Strong Base and Weak Base23:49
- Example 424:54
- Example 533:51
35m 34s
- Intro0:00
- Polyprotic Acids1:04
- Acids Dissociation1:05
- Example 14:51
- Example 217:30
- Example 331:11
41m 14s
- Intro0:00
- Salts and Their Acid-Base Properties0:11
- Salts and Their Acid-Base Properties0:15
- Example 17:58
- Example 214:00
- Metal Ion and Acidic Solution22:00
- Example 328:35
- NH₄F → NH₄⁺ + F⁻34:05
- Example 438:03
41m 58s
- Intro0:00
- Common Ion Effect & Buffers1:16
- Covalent Oxides Produce Acidic Solutions in Water1:36
- Ionic Oxides Produce Basic Solutions in Water4:15
- Practice Example 16:10
- Practice Example 29:00
- Definition12:27
- Example 1: Part A16:49
- Example 1: Part B19:54
- Buffer Solution25:10
- Example of Some Buffers: HF and NaF30:02
- Example of Some Buffers: Acetic Acid & Potassium Acetate31:34
- Example of Some Buffers: CH₃NH₂ & CH₃NH₃Cl33:54
- Example 2: Buffer Solution36:36
32m 24s
- Intro0:00
- Buffers1:20
- Buffer Solution1:21
- Adding Base5:03
- Adding Acid7:14
- Example 1: Question9:48
- Example 1: Recall12:08
- Example 1: Major Species Upon Addition of NaOH16:10
- Example 1: Equilibrium, ICE Chart, and Final Calculation24:33
- Example 1: Comparison29:19
40m 6s
- Intro0:00
- Buffers1:27
- Example 1: Question1:32
- Example 1: ICE Chart3:15
- Example 1: Major Species Upon Addition of OH⁻, But Before Rxn7:23
- Example 1: Equilibrium, ICE Chart, and Final Calculation12:51
- Summary17:21
- Another Look at Buffering & the Henderson-Hasselbalch equation19:00
- Example 227:08
- Example 332:01
38m 43s
- Intro0:00
- Buffers0:25
- Buffer Capacity Part 10:26
- Example 14:10
- Buffer Capacity Part 219:29
- Example 225:12
- Example 332:02
42m 42s
- Intro0:00
- Titrations: Strong Acid and Strong Base1:11
- Definition of Titration1:12
- Sample Problem3:33
- Definition of Titration Curve or pH Curve9:46
- Scenario 1: Strong Acid- Strong Base Titration11:00
- Question11:01
- Part 1: No NaOH is Added14:00
- Part 2: 10.0 mL of NaOH is Added15:50
- Part 3: Another 10.0 mL of NaOH & 20.0 mL of NaOH are Added22:19
- Part 4: 50.0 mL of NaOH is Added26:46
- Part 5: 100.0 mL (Total) of NaOH is Added27:26
- Part 6: 150.0 mL (Total) of NaOH is Added32:06
- Part 7: 200.0 mL of NaOH is Added35:07
- Titrations Curve for Strong Acid and Strong Base35:43
42m 3s
- Intro0:00
- Titrations: Weak Acid and Strong Base0:43
- Question0:44
- Part 1: No NaOH is Added1:54
- Part 2: 10.0 mL of NaOH is Added5:17
- Part 3: 25.0 mL of NaOH is Added14:01
- Part 4: 40.0 mL of NaOH is Added21:55
- Part 5: 50.0 mL (Total) of NaOH is Added22:25
- Part 6: 60.0 mL (Total) of NaOH is Added31:36
- Part 7: 75.0 mL (Total) of NaOH is Added35:44
- Titration Curve36:09
52m 3s
- Intro0:00
- Examples and Indicators0:25
- Example 1: Question0:26
- Example 1: Solution2:03
- Example 2: Question12:33
- Example 2: Solution14:52
- Example 3: Question23:45
- Example 3: Solution25:09
- Acid/Base Indicator Overview34:45
- Acid/Base Indicator Example37:40
- Acid/Base Indicator General Result47:11
- Choosing Acid/Base Indicator49:12
36m 25s
- Intro0:00
- Solubility Equilibria0:48
- Solubility Equilibria Overview0:49
- Solubility Product Constant4:24
- Definition of Solubility9:10
- Definition of Solubility Product11:28
- Example 114:09
- Example 220:19
- Example 327:30
- Relative Solubilities31:04
42m 6s
- Intro0:00
- Solubility Equilibria0:46
- Common Ion Effect0:47
- Example 13:14
- pH & Solubility13:00
- Example of pH & Solubility15:25
- Example 223:06
- Precipitation & Definition of the Ion Product26:48
- If Q > Ksp29:31
- If Q < Ksp30:27
- Example 332:58
43m 9s
- Intro0:00
- Solubility Equilibria0:55
- Example 1: Question0:56
- Example 1: Step 1 - Check to See if Anything Precipitates2:52
- Example 1: Step 2 - Stoichiometry10:47
- Example 1: Step 3 - Equilibrium16:34
- Example 2: Selective Precipitation (Question)21:02
- Example 2: Solution23:41
- Classical Qualitative Analysis29:44
- Groups: 1-538:44
43m 38s
- Intro0:00
- Complex Ion Equilibria0:32
- Complex Ion0:34
- Ligan Examples1:51
- Ligand Definition3:12
- Coordination6:28
- Example 18:08
- Example 219:13
31m 30s
- Intro0:00
- Complex Ions and Solubility0:23
- Recall: Classical Qualitative Analysis0:24
- Example 16:10
- Example 216:16
- Dissolving a Water-Insoluble Ionic Compound: Method 123:38
- Dissolving a Water-Insoluble Ionic Compound: Method 228:13
56m 28s
- Intro0:00
- Spontaneity, Entropy, Free Energy2:25
- Energy Overview2:26
- Equation: ∆E = q + w4:30
- State Function/ State Property8:35
- Equation: w = -P∆V12:00
- Enthalpy: H = E + PV14:50
- Enthalpy is a State Property17:33
- Exothermic and Endothermic Reactions19:20
- First Law of Thermodynamic22:28
- Entropy25:48
- Spontaneous Process33:53
- Second Law of Thermodynamic36:51
- More on Entropy42:23
- Example43:55
39m 55s
- Intro0:00
- Spontaneity, Entropy, Free Energy1:30
- ∆S of Universe = ∆S of System + ∆S of Surrounding1:31
- Convention3:32
- Examining a System5:36
- Thermodynamic Property: Sign of ∆S16:52
- Thermodynamic Property: Magnitude of ∆S18:45
- Deriving Equation: ∆S of Surrounding = -∆H / T20:25
- Example 125:51
- Free Energy Equations29:22
30m 10s
- Intro0:00
- Spontaneity, Entropy, Free Energy0:11
- Example 12:38
- Key Concept of Example 114:06
- Example 215:56
- Units for ∆H, ∆G, and S20:56
- ∆S of Surrounding & ∆S of System22:00
- Reaction Example24:17
- Example 326:52
30m 7s
- Intro0:00
- Spontaneity, Entropy, Free Energy0:29
- Standard Free Energy of Formation0:58
- Example 14:34
- Reaction Under Non-standard Conditions13:23
- Example 216:26
- ∆G = Negative22:12
- ∆G = 024:38
- Diagram Example of ∆G26:43
44m 56s
- Intro0:00
- Spontaneity, Entropy, Free Energy0:56
- Equations: ∆G of Reaction, ∆G°, and K0:57
- Example 1: Question6:50
- Example 1: Part A9:49
- Example 1: Part B15:28
- Example 217:33
- Example 323:31
- lnK = (- ∆H° ÷ R) ( 1 ÷ T) + ( ∆S° ÷ R)31:36
- Maximum Work35:57
39m 23s
- Intro0:00
- Oxidation-Reduction and Balancing2:06
- Definition of Electrochemistry2:07
- Oxidation and Reduction Review3:05
- Example 1: Assigning Oxidation State10:15
- Example 2: Is the Following a Redox Reaction?18:06
- Example 3: Step 1 - Write the Oxidation & Reduction Half Reactions22:46
- Example 3: Step 2 - Balance the Reaction26:44
- Example 3: Step 3 - Multiply30:11
- Example 3: Step 4 - Add32:07
- Example 3: Step 5 - Check33:29
43m 9s
- Intro0:00
- Galvanic Cells0:39
- Example 1: Balance the Following Under Basic Conditions0:40
- Example 1: Steps to Balance Reaction Under Basic Conditions3:25
- Example 1: Solution5:23
- Example 2: Balance the Following Reaction13:56
- Galvanic Cells18:15
- Example 3: Galvanic Cells28:19
- Example 4: Galvanic Cells35:12
48m 41s
- Intro0:00
- Cell Potential2:08
- Definition of Cell Potential2:17
- Symbol and Unit5:50
- Standard Reduction Potential10:16
- Example Figure 113:08
- Example Figure 219:00
- All Reduction Potentials are Written as Reduction23:10
- Cell Potential: Important Fact 126:49
- Cell Potential: Important Fact 227:32
- Cell Potential: Important Fact 328:54
- Cell Potential: Important Fact 430:05
- Example Problem 132:29
- Example Problem 238:38
41m 23s
- Intro0:00
- Potential, Work, Free Energy0:42
- Descriptions of Galvanic Cell0:43
- Line Notation5:33
- Example 16:26
- Example 211:15
- Example 315:18
- Equation: Volt22:20
- Equations: Cell Potential, Work, and Charge28:30
- Maximum Cell Potential is Related to the Free Energy of the Cell Reaction35:09
- Example 437:42
34m 19s
- Intro0:00
- Cell Potential & Concentration0:29
- Example 1: Question0:30
- Example 1: Nernst Equation4:43
- Example 1: Solution7:01
- Cell Potential & Concentration11:27
- Example 216:38
- Manipulating the Nernst Equation25:15
- Example 328:43
33m 21s
- Intro0:00
- Electrolysis3:16
- Electrolysis: Part 13:17
- Electrolysis: Part 25:25
- Galvanic Cell Example7:13
- Nickel Cadmium Battery12:18
- Ampere16:00
- Example 120:47
- Example 225:47
44m 45s
- Intro0:00
- Light2:14
- Introduction to Light2:15
- Frequency, Speed, and Wavelength of Waves3:58
- Units and Equations7:37
- Electromagnetic Spectrum12:13
- Example 1: Calculate the Frequency17:41
- E = hν21:30
- Example 2: Increment of Energy25:12
- Photon Energy of Light28:56
- Wave and Particle31:46
- Example 3: Wavelength of an Electron34:46
54m
- Intro0:00
- Quantum Mechanics & Electron Orbitals0:51
- Quantum Mechanics & Electron Orbitals Overview0:52
- Electron Orbital and Energy Levels for the Hydrogen Atom8:47
- Example 113:41
- Quantum Mechanics: Schrodinger Equation19:19
- Quantum Numbers Overview31:10
- Principal Quantum Numbers33:28
- Angular Momentum Numbers34:55
- Magnetic Quantum Numbers36:35
- Spin Quantum Numbers37:46
- Primary Level, Sublevels, and Sub-Sub-Levels39:42
- Example42:17
- Orbital & Quantum Numbers49:32
34m 4s
- Intro0:00
- Electron Configurations & Diagrams1:08
- Electronic Structure of Ground State Atom1:09
- Order of Electron Filling3:50
- Electron Configurations & Diagrams: H8:41
- Electron Configurations & Diagrams: He9:12
- Electron Configurations & Diagrams: Li9:47
- Electron Configurations & Diagrams: Be11:17
- Electron Configurations & Diagrams: B12:05
- Electron Configurations & Diagrams: C13:03
- Electron Configurations & Diagrams: N14:55
- Electron Configurations & Diagrams: O15:24
- Electron Configurations & Diagrams: F16:25
- Electron Configurations & Diagrams: Ne17:00
- Electron Configurations & Diagrams: S18:08
- Electron Configurations & Diagrams: Fe20:08
- Introduction to Valence Electrons23:04
- Valence Electrons of Oxygen23:44
- Valence Electrons of Iron24:02
- Valence Electrons of Arsenic24:30
- Valence Electrons: Exceptions25:36
- The Periodic Table27:52
52m 43s
- Intro0:00
- Vapor Pressure and Changes of State2:26
- Intermolecular Forces Overview2:27
- Hydrogen Bonding5:23
- Heat of Vaporization9:58
- Vapor Pressure: Definition and Example11:04
- Vapor Pressures is Mostly a Function of Intermolecular Forces17:41
- Vapor Pressure Increases with Temperature20:52
- Vapor Pressure vs. Temperature: Graph and Equation22:55
- Clausius-Clapeyron Equation31:55
- Example 132:13
- Heating Curve35:40
- Heat of Fusion41:31
- Example 243:45
31m 17s
- Intro0:00
- Phase Diagrams and Solutions0:22
- Definition of a Phase Diagram0:50
- Phase Diagram Part 1: H₂O1:54
- Phase Diagram Part 2: CO₂9:59
- Solutions: Solute & Solvent16:12
- Ways of Discussing Solution Composition: Mass Percent or Weight Percent18:46
- Ways of Discussing Solution Composition: Molarity20:07
- Ways of Discussing Solution Composition: Mole Fraction20:48
- Ways of Discussing Solution Composition: Molality21:41
- Example 1: Question22:06
- Example 1: Mass Percent24:32
- Example 1: Molarity25:53
- Example 1: Mole Fraction28:09
- Example 1: Molality29:36
37m 23s
- Intro0:00
- Vapor Pressure of Solutions2:07
- Vapor Pressure & Raoult's Law2:08
- Example 15:21
- When Ionic Compounds Dissolve10:51
- Example 212:38
- Non-Ideal Solutions17:42
- Negative Deviation24:23
- Positive Deviation29:19
- Example 331:40
34m 11s
- Intro0:00
- Colligative Properties1:07
- Boiling Point Elevation1:08
- Example 1: Question5:19
- Example 1: Solution6:52
- Freezing Point Depression12:01
- Example 2: Question14:46
- Example 2: Solution16:34
- Osmotic Pressure20:20
- Example 3: Question28:00
- Example 3: Solution30:16
48m 39s
- Intro0:00
- Bonding & Lewis Structure2:23
- Covalent Bond2:24
- Single Bond, Double Bond, and Triple Bond4:11
- Bond Length & Intermolecular Distance5:51
- Definition of Electronegativity8:42
- Bond Polarity11:48
- Bond Energy20:04
- Example 124:31
- Definition of Lewis Structure31:54
- Steps in Forming a Lewis Structure33:26
- Lewis Structure Example: H₂36:53
- Lewis Structure Example: CH₄37:33
- Lewis Structure Example: NO⁺38:43
- Lewis Structure Example: PCl₅41:12
- Lewis Structure Example: ICl₄⁻43:05
- Lewis Structure Example: BeCl₂45:07
36m 59s
- Intro0:00
- Resonance and Formal Charge0:09
- Resonance Structures of NO₃⁻0:25
- Resonance Structures of NO₂⁻12:28
- Resonance Structures of HCO₂⁻16:28
- Formal Charge19:40
- Formal Charge Example: SO₄²⁻21:32
- Formal Charge Example: CO₂31:33
- Formal Charge Example: HCN32:44
- Formal Charge Example: CN⁻33:34
- Formal Charge Example: 0₃34:43
41m 21s
- Intro0:00
- Shapes of Molecules0:35
- VSEPR0:36
- Steps in Determining Shapes of Molecules6:18
- Linear11:38
- Trigonal Planar11:55
- Tetrahedral12:45
- Trigonal Bipyramidal13:23
- Octahedral14:29
- Table: Shapes of Molecules15:40
- Example: CO₂21:11
- Example: NO₃⁻24:01
- Example: H₂O27:00
- Example: NH₃29:48
- Example: PCl₃⁻32:18
- Example: IF₄⁺34:38
- Example: KrF₄37:57
40m 17s
- Intro0:00
- Hybrid Orbitals0:13
- Introduction to Hybrid Orbitals0:14
- Electron Orbitals for CH₄5:02
- sp³ Hybridization10:52
- Example: sp³ Hybridization12:06
- sp² Hybridization14:21
- Example: sp² Hybridization16:11
- σ Bond19:10
- π Bond20:07
- sp Hybridization & Example22:00
- dsp³ Hybridization & Example27:36
- d²sp³ Hybridization & Example30:36
- Example: Predict the Hybridization and Describe the Molecular Geometry of CO32:31
- Example: Predict the Hybridization and Describe the Molecular Geometry of BF₄⁻35:17
- Example: Predict the Hybridization and Describe the Molecular Geometry of XeF₂37:09
52m 34s
- Intro0:00
- Multiple Choice1:21
- Multiple Choice 11:22
- Multiple Choice 22:23
- Multiple Choice 33:38
- Multiple Choice 44:34
- Multiple Choice 55:16
- Multiple Choice 65:41
- Multiple Choice 76:20
- Multiple Choice 87:03
- Multiple Choice 97:31
- Multiple Choice 109:03
- Multiple Choice 1111:52
- Multiple Choice 1213:16
- Multiple Choice 1313:56
- Multiple Choice 1414:52
- Multiple Choice 1515:43
- Multiple Choice 1616:20
- Multiple Choice 1716:55
- Multiple Choice 1817:22
- Multiple Choice 1918:59
- Multiple Choice 2020:24
- Multiple Choice 2122:20
- Multiple Choice 2223:29
- Multiple Choice 2324:30
- Multiple Choice 2425:24
- Multiple Choice 2526:21
- Multiple Choice 2629:06
- Multiple Choice 2730:42
- Multiple Choice 2833:28
- Multiple Choice 2934:38
- Multiple Choice 3035:37
- Multiple Choice 3137:31
- Multiple Choice 3238:28
- Multiple Choice 3339:50
- Multiple Choice 3442:57
- Multiple Choice 3544:18
- Multiple Choice 3645:52
- Multiple Choice 3748:02
- Multiple Choice 3849:25
- Multiple Choice 3949:43
- Multiple Choice 4050:16
- Multiple Choice 4150:49
32m 15s
- Intro0:00
- Multiple Choice0:12
- Multiple Choice 420:13
- Multiple Choice 430:33
- Multiple Choice 441:16
- Multiple Choice 452:36
- Multiple Choice 465:22
- Multiple Choice 476:35
- Multiple Choice 488:02
- Multiple Choice 4910:05
- Multiple Choice 5010:26
- Multiple Choice 5111:07
- Multiple Choice 5212:01
- Multiple Choice 5312:55
- Multiple Choice 5416:12
- Multiple Choice 5518:11
- Multiple Choice 5619:45
- Multiple Choice 5720:15
- Multiple Choice 5823:28
- Multiple Choice 5924:27
- Multiple Choice 6026:45
- Multiple Choice 6129:15
32m 50s
- Intro0:00
- Multiple Choice0:16
- Multiple Choice 620:17
- Multiple Choice 631:57
- Multiple Choice 646:16
- Multiple Choice 658:05
- Multiple Choice 669:18
- Multiple Choice 6710:38
- Multiple Choice 6812:51
- Multiple Choice 6914:32
- Multiple Choice 7017:35
- Multiple Choice 7122:44
- Multiple Choice 7224:27
- Multiple Choice 7327:46
- Multiple Choice 7429:39
- Multiple Choice 7530:23
47m 22s
- Intro0:00
- Free Response0:15
- Free Response 1: Part A0:16
- Free Response 1: Part B4:15
- Free Response 1: Part C5:47
- Free Response 1: Part D9:20
- Free Response 1: Part E. i10:58
- Free Response 1: Part E. ii16:45
- Free Response 1: Part E. iii26:03
- Free Response 2: Part A. i31:01
- Free Response 2: Part A. ii33:38
- Free Response 2: Part A. iii35:20
- Free Response 2: Part B. i37:38
- Free Response 2: Part B. ii39:30
- Free Response 2: Part B. iii44:44
43m 5s
- Intro0:00
- Free Response0:12
- Free Response 3: Part A0:13
- Free Response 3: Part B6:25
- Free Response 3: Part C. i11:33
- Free Response 3: Part C. ii12:02
- Free Response 3: Part D14:30
- Free Response 4: Part A21:03
- Free Response 4: Part B22:59
- Free Response 4: Part C24:33
- Free Response 4: Part D27:22
- Free Response 4: Part E28:43
- Free Response 4: Part F29:35
- Free Response 4: Part G30:15
- Free Response 4: Part H30:48
- Free Response 5: Diagram32:00
- Free Response 5: Part A34:14
- Free Response 5: Part B36:07
- Free Response 5: Part C37:45
- Free Response 5: Part D39:00
- Free Response 5: Part E40:26
28m 36s
- Intro0:00
- Free Response0:43
- Free Response 6: Part A. i0:44
- Free Response 6: Part A. ii3:08
- Free Response 6: Part A. iii5:02
- Free Response 6: Part B. i7:11
- Free Response 6: Part B. ii9:40
- Free Response 7: Part A11:14
- Free Response 7: Part B13:45
- Free Response 7: Part C15:43
- Free Response 7: Part D16:54
- Free Response 8: Part A. i19:15
- Free Response 8: Part A. ii21:16
- Free Response 8: Part B. i23:51
- Free Response 8: Part B. ii25:07
For more information, please see full course syllabus of AP Chemistry
AP Chemistry Spontaneity, Entropy, & Free Energy, Part I
Heat, energy, entropy, and spontaneity are all core concepts of thermodynamics. This lecture reviews the basic formulas for heat and work as well as the first and second laws of thermodynamics. The first law of thermodynamics is the conservation of energy. Energy in the universe is constant and cannot be created or destroyed. It can only change form (between heat and work). Spontaneous processes take place without outside intervention. The second law of thermodynamics states that spontaneous processes increase the entropy of the universe. Chaotic, random systems with many possible configurations have a higher entropy than orderly systems.
Share this knowledge with your friends!
Copy & Paste this embed code into your website’s HTML
Please ensure that your website editor is in text mode when you paste the code.(In Wordpress, the mode button is on the top right corner.)
- - Allow users to view the embedded video in full-size.
2 answers
Last reply by: Parth Shorey
Fri Oct 30, 2015 2:23 PM
Post by Parth Shorey on October 29, 2015
Another question, was the confusion regarding W and Q. You had said Work done by the system is - but considering that I am preparing for my MCATs, I was looking into my physics textbook and it says the opposite of what you said. That work is positive done by the system rather than on it. I was confused because it doesn't make sense to me.
1 answer
Thu Oct 29, 2015 10:49 PM
Post by Parth Shorey on October 29, 2015
Why did you use W=-PV and not PV?
1 answer
Sat Oct 24, 2015 6:46 PM
Post by Jason Smith on October 24, 2015
Hi professor, when you say that the probability of finding a gas bunched together in a corner is zero, do you actually mean zero? Or do you mean highly unlikely? For example, I understand that gasses expand because there are more "states" that it exists in this way compared to a bunched together state. However, given say, 10 trillion years, could a gas EVER spontaneously bunch back together? 100 trillion years? 100 trillion x 100 trillion years? I find this topic very fascinating and I have lots of questions about it. Thank you professor.
1 answer
Sun Oct 18, 2015 11:55 PM
Post by Jason Smith on October 15, 2015
I like to imagine state functions being like this: imagine having a graph chart (sort of like you have in geometry). Now, imagine there being two dots on different locations on the chart. No matter what route you take, the total number is always gonna be the same! Even if I went completely crazy zig-zagging throughout the graph, I would eventually end up with the same number once I reached the second dot. Intuitively, this has helped me make sense of state functions.
2 answers
Last reply by: Jason Smith
Mon Oct 19, 2015 4:58 PM
Post by Jason Smith on October 15, 2015
I can always assume that if q is positive than w will always be negative? Does q and w always end up summing up to zero? Thanks professor.
2 answers
Fri Mar 13, 2015 4:12 AM
Post by Danial Shadmany on March 13, 2015
Why is heat not a state property but Enthalpy is. Aren't they similar?
2 answers
Last reply by: David Gonzalez
Mon Jan 12, 2015 12:56 PM
Post by David Gonzalez on January 12, 2015
Hi professor, great lecture!
I was sitting down yesterday pondering about science, and I was curious as to what your opinion might be to this question: do you think we've learned everything there is to know about thermodynamics? For instance, is there some mysterious "thing" that we don't know yet that might change these principles? Or are these pretty much going to be the same, say, 200 years from now?
Thanks professor.
1 answer
Fri Apr 4, 2014 6:54 PM
Post by Mohamed Kaba on April 4, 2014
This makes too much sense. Thank you sir. I wish that I had discovered your Chem. playlist earlier. I've traveled to the far reaches of google search results(page 10+) to understand entropy, but in a few minutes of watching these videos everything suddenly makes sense.
1 answer
Fri Jul 12, 2013 4:08 PM
Post by KyungYeop Kim on July 11, 2013
(Regarding your kind post on Facebook) thank you very much sir, the concept is much clearer to me now due to your lucid explication. I don't think I'll ever struggle with it again, given that I now finally understand it! May I ask one last question(at least for a while) relating to the further discourse on spontaneity? Suppose there's an exothermic equation and its value of ∆H is some negative number that doesn't matter at the moment. I am asked to show how the temperature, at which the reaction changes from nonspontenous to spontenous, can be predicted. Of course I flipped the equation and got T=(∆G-∆H)/∆S to legitimately calculate the T, and determined that I'd have to know both ∆G and ∆S to do that. But the answer is such that only the value of ∆S seems necessary; Would you agree that both ∆G and ∆S are needed for the calculation regarding the change from non-spontaneous to spontaneous? if so, why? (Please not that there's no other information available; I think unless there's some unknown way to calculate ∆G, then I may be right)Thank you.
1 answer
Wed Jul 10, 2013 7:42 PM
Post by KyungYeop Kim on July 9, 2013
I understand that ∆H-T∆S<0, but I still don't get the charges of ∆H and T∆S and why they have to be that way. Could you provide the document if you would be so nice? I will certainly try to understand but it seems any good resource might help me greatly. Thank you again..
3 answers
Last reply by: KyungYeop Kim
Tue Jul 9, 2013 9:58 PM
Post by KyungYeop Kim on July 9, 2013
Regarding a specific situation in which a nonspontaneous reaction under standard conditions becomes spontaneous at lower temperature, how can I describe this phenomenon in relation to enthalpy, entropy, and free energy? and how can I explain it in terms of temperature change? I've succeeded so far in determining that ΔG>0 since it's nonspontaneous under standard conditions, but what about ΔH and ΔS?
Given the equation = ΔG = ΔH*TΔS; I think the fact that temperature(T) can go either from positive to negative or negative to negative seems to confuse me. Are we assuming, in saying lowering temperature, that the T goes from negative(-) to negative(-)?
I know it's a complex problem, and I apologize if I'm asking too much, but I would like to know what the answer is and why. Thank you always!
1 answer
Mon Apr 15, 2013 7:39 PM
Post by William Dawson on April 14, 2013
Isn't it better to define entropy as the measure of the statistical liklihood of a given configuration, in relation to the total possible number of configurations for that system? Calling it a 'measure of disroder' or of chaos leaves too much language to be interpreted, when really it's a statistical reality best defined with direct reference to the math and not to the symantics of potentially loaded words.
1 answer
Sun Apr 14, 2013 1:20 PM
Post by carlos bara on April 14, 2013
Professor Hovasapian, why is entropy abbreviated with the letter S? what does the s stand for?
2 answers
Fri Feb 15, 2013 5:10 AM
Post by Tong Lai on February 11, 2013
Thank you professor, I like the way you do your lectures. You explain everything and try to keep everything simple for me to understand. Some other professors ,I have to say, try to make everything complex...I will say u are the best professor here in educator.com. Thanks.
1 answer
Thu Oct 18, 2012 4:38 PM
Post by Carina Tull on October 18, 2012
Great Lecture! Thank you very much
1 answer
Sun Oct 7, 2012 2:39 PM
Post by Riley Argue on October 6, 2012
Thank you Professor Hovasapian!
Excellent work as always!
1 answer
Sat Jul 14, 2012 9:09 PM
Post by Vinh Dong on June 5, 2012
Thank you for your awesome lectures. May I say, you look like Albert Einstein. No offense. :P