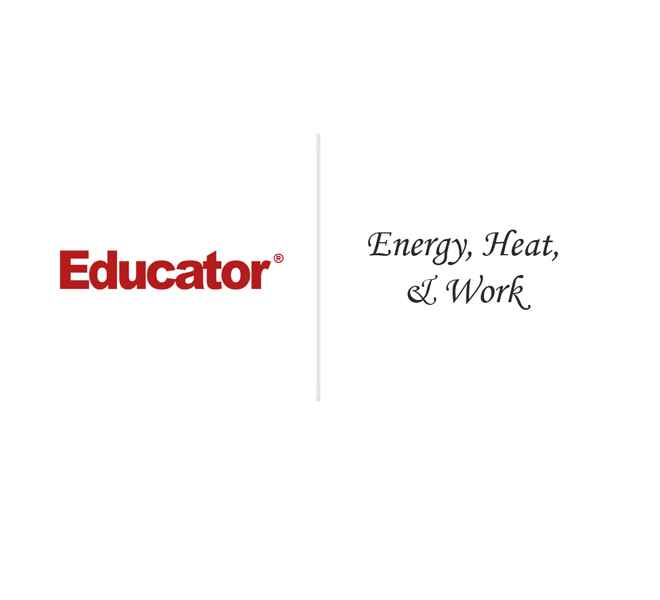
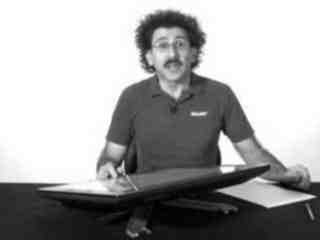
Raffi Hovasapian
Energy, Heat, and Work
Slide Duration:Table of Contents
41m 24s
- Intro0:00
- Periodic Table of Elements0:15
- Naming Compounds3:13
- Definition and Examples of Ions3:14
- Ionic (Symbol to Name): NaCl5:23
- Ionic (Name to Symbol): Calcium Oxide7:58
- Ionic - Polyatoms Anions: Examples12:45
- Ionic - Polyatoms Anions (Symbol to Name): KClO14:50
- Ionic - Polyatoms Anions (Name to Symbol): Potassium Phosphate15:49
- Ionic Compounds Involving Transition Metals (Symbol to Name): Co₂(CO₃)₃20:48
- Ionic Compounds Involving Transition Metals (Name to Symbol): Palladium 2 Acetate22:44
- Naming Covalent Compounds (Symbol to Name): CO26:21
- Naming Covalent Compounds (Name to Symbol): Nitrogen Trifluoride27:34
- Naming Covalent Compounds (Name to Symbol): Dichlorine Monoxide27:57
- Naming Acids Introduction28:11
- Naming Acids (Name to Symbol): Chlorous Acid35:08
- % Composition by Mass Example37:38
37m 19s
- Intro0:00
- Stoichiometry0:25
- Introduction to Stoichiometry0:26
- Example 15:03
- Example 210:17
- Example 315:09
- Example 424:02
- Example 5: Questions28:11
- Example 5: Part A - Limiting Reactant30:30
- Example 5: Part B32:27
- Example 5: Part C35:00
31m 14s
- Intro0:00
- Precipitation Reactions0:53
- Dissociation of ionic Compounds0:54
- Solubility Guidelines for ionic Compounds: Soluble Ionic Compounds8:15
- Solubility Guidelines for ionic Compounds: Insoluble ionic Compounds12:56
- Precipitation Reactions14:08
- Example 1: Mixing a Solution of BaCl₂ & K₂SO₄21:21
- Example 2: Mixing a Solution of Mg(NO₃)₂ & KI26:10
43m 21s
- Intro0:00
- Acid-Base Reactions1:00
- Introduction to Acid: Monoprotic Acid and Polyprotic Acid1:01
- Introduction to Base8:28
- Neutralization11:45
- Example 116:17
- Example 221:55
- Molarity24:50
- Example 326:50
- Example 430:01
- Example 4: Limiting Reactant37:51
- Example 4: Reaction Part40:01
47m 58s
- Intro0:00
- Oxidation Reduction Reactions0:26
- Oxidation and Reduction Overview0:27
- How Can One Tell Whether Oxidation-Reduction has Taken Place?7:13
- Rules for Assigning Oxidation State: Number 111:22
- Rules for Assigning Oxidation State: Number 212:46
- Rules for Assigning Oxidation State: Number 313:25
- Rules for Assigning Oxidation State: Number 414:50
- Rules for Assigning Oxidation State: Number 515:41
- Rules for Assigning Oxidation State: Number 617:00
- Example 1: Determine the Oxidation State of Sulfur in the Following Compounds18:20
- Activity Series and Reduction Properties25:32
- Activity Series and Reduction Properties25:33
- Example 2: Write the Balance Molecular, Total Ionic, and Net Ionic Equations for Al + HCl31:37
- Example 334:25
- Example 437:55
31m 50s
- Intro0:00
- Stoichiometry Example 10:36
- Example 1: Question and Answer0:37
- Stoichiometry Example 26:57
- Example 2: Questions6:58
- Example 2: Part A Solution12:16
- Example 2: Part B Solution13:05
- Example 2: Part C Solution14:00
- Example 2: Part D Solution14:38
- Stoichiometry Example 317:56
- Example 3: Questions17:57
- Example 3: Part A Solution19:51
- Example 3: Part B Solution21:43
- Example 3: Part C Solution26:46
49m 40s
- Intro0:00
- Pressure0:22
- Pressure Overview0:23
- Torricelli: Barometer4:35
- Measuring Gas Pressure in a Container7:49
- Boyle's Law12:40
- Example 116:56
- Gas Laws21:18
- Gas Laws21:19
- Avogadro's Law26:16
- Example 231:47
- Ideal Gas Equation38:20
- Standard Temperature and Pressure (STP)38:21
- Example 340:43
32m
- Intro0:00
- Gases0:27
- Gases0:28
- Mole Fractions5:52
- Vapor Pressure8:22
- Example 113:25
- Example 222:45
31m 58s
- Intro0:00
- Kinetic Molecular Theory and Real Gases0:45
- Kinetic Molecular Theory 10:46
- Kinetic Molecular Theory 24:23
- Kinetic Molecular Theory 35:42
- Kinetic Molecular Theory 46:27
- Equations7:52
- Effusion11:15
- Diffusion13:30
- Example 119:54
- Example 223:23
- Example 326:45
25m 34s
- Intro0:00
- Example 10:34
- Example 10:35
- Example 26:15
- Example 2: Part A6:16
- Example 2: Part B8:46
- Example 2: Part C10:30
- Example 2: Part D11:15
- Example 2: Part E12:20
- Example 2: Part F13:22
- Example 314:45
- Example 314:46
- Example 418:16
- Example 418:17
- Example 521:04
- Example 521:05
37m 32s
- Intro0:00
- Thermochemistry0:25
- Temperature and Heat0:26
- Work3:07
- System, Surroundings, Exothermic Process, and Endothermic Process8:19
- Work & Gas: Expansion and Compression16:30
- Example 124:41
- Example 227:47
- Example 331:58
32m 34s
- Intro0:00
- Thermochemistry1:43
- Defining Enthalpy & Hess's Law1:44
- Example 16:48
- State Function13:11
- Example 217:15
- Example 324:09
23m 9s
- Intro0:00
- Thermochemistry1:04
- Standard Enthalpy of Formation: Definition & Equation1:05
- ∆H of Formation10:00
- Example 111:22
- Example 219:00
39m 28s
- Intro0:00
- Thermochemistry0:21
- Heat Capacity0:22
- Molar Heat Capacity4:44
- Constant Pressure Calorimetry5:50
- Example 112:24
- Constant Volume Calorimetry21:54
- Example 224:40
- Example 331:03
36m 24s
- Intro0:00
- Kinetics2:18
- Rate: 2 NO₂ (g) → 2NO (g) + O₂ (g)2:19
- Reaction Rates Graph7:25
- Time Interval & Average Rate13:13
- Instantaneous Rate15:13
- Rate of Reaction is Proportional to Some Power of the Reactant Concentrations23:49
- Example 127:19
30m 48s
- Intro0:00
- Kinetics0:33
- Rate0:34
- Idea2:24
- Example 1: NH₄⁺ + NO₂⁻ → NO₂ (g) + 2 H₂O5:36
- Example 2: BrO₃⁻ + 5 Br⁻ + 6 H⁺ → 3 Br₂ + 3 H₂O19:29
32m 17s
- Intro0:00
- Kinetics0:52
- Integrated Rate Law0:53
- Example 16:26
- Example 215:19
- Half-life of a Reaction20:40
- Example 3: Part A25:41
- Example 3: Part B28:01
26m 40s
- Intro0:00
- Kinetics0:22
- Second Order0:23
- Example 16:08
- Zero-Order16:36
- Summary for the Kinetics Associated with the Reaction21:27
40m 59s
- Intro0:00
- Kinetics0:53
- Rate Constant0:54
- Collision Model2:45
- Activation Energy5:11
- Arrhenius Proposed9:54
- 2 Requirements for a Successful Reaction15:39
- Rate Constant17:53
- Arrhenius Equation19:51
- Example 125:00
- Activation Energy & the Values of K32:12
- Example 236:46
29m 8s
- Intro0:00
- Kinetics0:43
- Example 10:44
- Example 26:53
- Example 38:58
- Example 411:36
- Example 516:36
- Example 6: Part A21:00
- Example 6: Part B25:09
46m
- Intro0:00
- Equilibrium1:32
- Introduction to Equilibrium1:33
- Equilibrium Rules14:00
- Example 1: Part A16:46
- Example 1: Part B18:48
- Example 1: Part C22:13
- Example 1: Part D24:55
- Example 2: Part A27:46
- Example 2: Part B31:22
- Example 2: Part C33:00
- Reverse a Reaction36:04
- Example 337:24
40m 53s
- Intro0:00
- Equilibrium1:31
- Equilibriums Involving Gases1:32
- General Equation10:11
- Example 1: Question11:55
- Example 1: Answer13:43
- Example 2: Question19:08
- Example 2: Answer21:37
- Example 3: Question33:40
- Example 3: Answer35:24
45m 53s
- Intro0:00
- Equilibrium0:57
- Reaction Quotient0:58
- If Q > K5:37
- If Q < K6:52
- If Q = K7:45
- Example 1: Part A8:24
- Example 1: Part B13:11
- Example 2: Question20:04
- Example 2: Answer22:15
- Example 3: Question30:54
- Example 3: Answer32:52
- Steps in Solving Equilibrium Problems42:40
31m 51s
- Intro0:00
- Equilibrium1:09
- Example 1: Question1:10
- Example 1: Answer4:15
- Example 2: Question13:04
- Example 2: Answer15:20
- Example 3: Question25:03
- Example 3: Answer26:32
40m 52s
- Intro0:00
- Le Chatelier1:05
- Le Chatelier Principle1:06
- Concentration: Add 'x'5:25
- Concentration: Subtract 'x'7:50
- Example 19:44
- Change in Pressure12:53
- Example 220:40
- Temperature: Exothermic and Endothermic24:33
- Example 329:55
- Example 435:30
50m 11s
- Intro0:00
- Acids and Bases1:14
- Bronsted-Lowry Acid-Base Model1:28
- Reaction of an Acid with Water4:36
- Acid Dissociation10:51
- Acid Strength13:48
- Example 121:22
- Water as an Acid & a Base25:25
- Example 2: Part A32:30
- Example 2: Part B34:47
- Example 3: Part A35:58
- Example 3: Part B39:33
- pH Scale41:12
- Example 443:56
43m 52s
- Intro0:00
- pH of Weak Acid Solutions1:12
- pH of Weak Acid Solutions1:13
- Example 16:26
- Example 214:25
- Example 324:23
- Example 430:38
43m 4s
- Intro0:00
- Bases0:33
- Percent Dissociation: Strong & Weak Bases0:45
- Example 16:23
- Strong Base Dissociation11:24
- Example 213:02
- Weak Acid and General Reaction17:38
- Example: NaOH → Na⁺ + OH⁻20:30
- Strong Base and Weak Base23:49
- Example 424:54
- Example 533:51
35m 34s
- Intro0:00
- Polyprotic Acids1:04
- Acids Dissociation1:05
- Example 14:51
- Example 217:30
- Example 331:11
41m 14s
- Intro0:00
- Salts and Their Acid-Base Properties0:11
- Salts and Their Acid-Base Properties0:15
- Example 17:58
- Example 214:00
- Metal Ion and Acidic Solution22:00
- Example 328:35
- NH₄F → NH₄⁺ + F⁻34:05
- Example 438:03
41m 58s
- Intro0:00
- Common Ion Effect & Buffers1:16
- Covalent Oxides Produce Acidic Solutions in Water1:36
- Ionic Oxides Produce Basic Solutions in Water4:15
- Practice Example 16:10
- Practice Example 29:00
- Definition12:27
- Example 1: Part A16:49
- Example 1: Part B19:54
- Buffer Solution25:10
- Example of Some Buffers: HF and NaF30:02
- Example of Some Buffers: Acetic Acid & Potassium Acetate31:34
- Example of Some Buffers: CH₃NH₂ & CH₃NH₃Cl33:54
- Example 2: Buffer Solution36:36
32m 24s
- Intro0:00
- Buffers1:20
- Buffer Solution1:21
- Adding Base5:03
- Adding Acid7:14
- Example 1: Question9:48
- Example 1: Recall12:08
- Example 1: Major Species Upon Addition of NaOH16:10
- Example 1: Equilibrium, ICE Chart, and Final Calculation24:33
- Example 1: Comparison29:19
40m 6s
- Intro0:00
- Buffers1:27
- Example 1: Question1:32
- Example 1: ICE Chart3:15
- Example 1: Major Species Upon Addition of OH⁻, But Before Rxn7:23
- Example 1: Equilibrium, ICE Chart, and Final Calculation12:51
- Summary17:21
- Another Look at Buffering & the Henderson-Hasselbalch equation19:00
- Example 227:08
- Example 332:01
38m 43s
- Intro0:00
- Buffers0:25
- Buffer Capacity Part 10:26
- Example 14:10
- Buffer Capacity Part 219:29
- Example 225:12
- Example 332:02
42m 42s
- Intro0:00
- Titrations: Strong Acid and Strong Base1:11
- Definition of Titration1:12
- Sample Problem3:33
- Definition of Titration Curve or pH Curve9:46
- Scenario 1: Strong Acid- Strong Base Titration11:00
- Question11:01
- Part 1: No NaOH is Added14:00
- Part 2: 10.0 mL of NaOH is Added15:50
- Part 3: Another 10.0 mL of NaOH & 20.0 mL of NaOH are Added22:19
- Part 4: 50.0 mL of NaOH is Added26:46
- Part 5: 100.0 mL (Total) of NaOH is Added27:26
- Part 6: 150.0 mL (Total) of NaOH is Added32:06
- Part 7: 200.0 mL of NaOH is Added35:07
- Titrations Curve for Strong Acid and Strong Base35:43
42m 3s
- Intro0:00
- Titrations: Weak Acid and Strong Base0:43
- Question0:44
- Part 1: No NaOH is Added1:54
- Part 2: 10.0 mL of NaOH is Added5:17
- Part 3: 25.0 mL of NaOH is Added14:01
- Part 4: 40.0 mL of NaOH is Added21:55
- Part 5: 50.0 mL (Total) of NaOH is Added22:25
- Part 6: 60.0 mL (Total) of NaOH is Added31:36
- Part 7: 75.0 mL (Total) of NaOH is Added35:44
- Titration Curve36:09
52m 3s
- Intro0:00
- Examples and Indicators0:25
- Example 1: Question0:26
- Example 1: Solution2:03
- Example 2: Question12:33
- Example 2: Solution14:52
- Example 3: Question23:45
- Example 3: Solution25:09
- Acid/Base Indicator Overview34:45
- Acid/Base Indicator Example37:40
- Acid/Base Indicator General Result47:11
- Choosing Acid/Base Indicator49:12
36m 25s
- Intro0:00
- Solubility Equilibria0:48
- Solubility Equilibria Overview0:49
- Solubility Product Constant4:24
- Definition of Solubility9:10
- Definition of Solubility Product11:28
- Example 114:09
- Example 220:19
- Example 327:30
- Relative Solubilities31:04
42m 6s
- Intro0:00
- Solubility Equilibria0:46
- Common Ion Effect0:47
- Example 13:14
- pH & Solubility13:00
- Example of pH & Solubility15:25
- Example 223:06
- Precipitation & Definition of the Ion Product26:48
- If Q > Ksp29:31
- If Q < Ksp30:27
- Example 332:58
43m 9s
- Intro0:00
- Solubility Equilibria0:55
- Example 1: Question0:56
- Example 1: Step 1 - Check to See if Anything Precipitates2:52
- Example 1: Step 2 - Stoichiometry10:47
- Example 1: Step 3 - Equilibrium16:34
- Example 2: Selective Precipitation (Question)21:02
- Example 2: Solution23:41
- Classical Qualitative Analysis29:44
- Groups: 1-538:44
43m 38s
- Intro0:00
- Complex Ion Equilibria0:32
- Complex Ion0:34
- Ligan Examples1:51
- Ligand Definition3:12
- Coordination6:28
- Example 18:08
- Example 219:13
31m 30s
- Intro0:00
- Complex Ions and Solubility0:23
- Recall: Classical Qualitative Analysis0:24
- Example 16:10
- Example 216:16
- Dissolving a Water-Insoluble Ionic Compound: Method 123:38
- Dissolving a Water-Insoluble Ionic Compound: Method 228:13
56m 28s
- Intro0:00
- Spontaneity, Entropy, Free Energy2:25
- Energy Overview2:26
- Equation: ∆E = q + w4:30
- State Function/ State Property8:35
- Equation: w = -P∆V12:00
- Enthalpy: H = E + PV14:50
- Enthalpy is a State Property17:33
- Exothermic and Endothermic Reactions19:20
- First Law of Thermodynamic22:28
- Entropy25:48
- Spontaneous Process33:53
- Second Law of Thermodynamic36:51
- More on Entropy42:23
- Example43:55
39m 55s
- Intro0:00
- Spontaneity, Entropy, Free Energy1:30
- ∆S of Universe = ∆S of System + ∆S of Surrounding1:31
- Convention3:32
- Examining a System5:36
- Thermodynamic Property: Sign of ∆S16:52
- Thermodynamic Property: Magnitude of ∆S18:45
- Deriving Equation: ∆S of Surrounding = -∆H / T20:25
- Example 125:51
- Free Energy Equations29:22
30m 10s
- Intro0:00
- Spontaneity, Entropy, Free Energy0:11
- Example 12:38
- Key Concept of Example 114:06
- Example 215:56
- Units for ∆H, ∆G, and S20:56
- ∆S of Surrounding & ∆S of System22:00
- Reaction Example24:17
- Example 326:52
30m 7s
- Intro0:00
- Spontaneity, Entropy, Free Energy0:29
- Standard Free Energy of Formation0:58
- Example 14:34
- Reaction Under Non-standard Conditions13:23
- Example 216:26
- ∆G = Negative22:12
- ∆G = 024:38
- Diagram Example of ∆G26:43
44m 56s
- Intro0:00
- Spontaneity, Entropy, Free Energy0:56
- Equations: ∆G of Reaction, ∆G°, and K0:57
- Example 1: Question6:50
- Example 1: Part A9:49
- Example 1: Part B15:28
- Example 217:33
- Example 323:31
- lnK = (- ∆H° ÷ R) ( 1 ÷ T) + ( ∆S° ÷ R)31:36
- Maximum Work35:57
39m 23s
- Intro0:00
- Oxidation-Reduction and Balancing2:06
- Definition of Electrochemistry2:07
- Oxidation and Reduction Review3:05
- Example 1: Assigning Oxidation State10:15
- Example 2: Is the Following a Redox Reaction?18:06
- Example 3: Step 1 - Write the Oxidation & Reduction Half Reactions22:46
- Example 3: Step 2 - Balance the Reaction26:44
- Example 3: Step 3 - Multiply30:11
- Example 3: Step 4 - Add32:07
- Example 3: Step 5 - Check33:29
43m 9s
- Intro0:00
- Galvanic Cells0:39
- Example 1: Balance the Following Under Basic Conditions0:40
- Example 1: Steps to Balance Reaction Under Basic Conditions3:25
- Example 1: Solution5:23
- Example 2: Balance the Following Reaction13:56
- Galvanic Cells18:15
- Example 3: Galvanic Cells28:19
- Example 4: Galvanic Cells35:12
48m 41s
- Intro0:00
- Cell Potential2:08
- Definition of Cell Potential2:17
- Symbol and Unit5:50
- Standard Reduction Potential10:16
- Example Figure 113:08
- Example Figure 219:00
- All Reduction Potentials are Written as Reduction23:10
- Cell Potential: Important Fact 126:49
- Cell Potential: Important Fact 227:32
- Cell Potential: Important Fact 328:54
- Cell Potential: Important Fact 430:05
- Example Problem 132:29
- Example Problem 238:38
41m 23s
- Intro0:00
- Potential, Work, Free Energy0:42
- Descriptions of Galvanic Cell0:43
- Line Notation5:33
- Example 16:26
- Example 211:15
- Example 315:18
- Equation: Volt22:20
- Equations: Cell Potential, Work, and Charge28:30
- Maximum Cell Potential is Related to the Free Energy of the Cell Reaction35:09
- Example 437:42
34m 19s
- Intro0:00
- Cell Potential & Concentration0:29
- Example 1: Question0:30
- Example 1: Nernst Equation4:43
- Example 1: Solution7:01
- Cell Potential & Concentration11:27
- Example 216:38
- Manipulating the Nernst Equation25:15
- Example 328:43
33m 21s
- Intro0:00
- Electrolysis3:16
- Electrolysis: Part 13:17
- Electrolysis: Part 25:25
- Galvanic Cell Example7:13
- Nickel Cadmium Battery12:18
- Ampere16:00
- Example 120:47
- Example 225:47
44m 45s
- Intro0:00
- Light2:14
- Introduction to Light2:15
- Frequency, Speed, and Wavelength of Waves3:58
- Units and Equations7:37
- Electromagnetic Spectrum12:13
- Example 1: Calculate the Frequency17:41
- E = hν21:30
- Example 2: Increment of Energy25:12
- Photon Energy of Light28:56
- Wave and Particle31:46
- Example 3: Wavelength of an Electron34:46
54m
- Intro0:00
- Quantum Mechanics & Electron Orbitals0:51
- Quantum Mechanics & Electron Orbitals Overview0:52
- Electron Orbital and Energy Levels for the Hydrogen Atom8:47
- Example 113:41
- Quantum Mechanics: Schrodinger Equation19:19
- Quantum Numbers Overview31:10
- Principal Quantum Numbers33:28
- Angular Momentum Numbers34:55
- Magnetic Quantum Numbers36:35
- Spin Quantum Numbers37:46
- Primary Level, Sublevels, and Sub-Sub-Levels39:42
- Example42:17
- Orbital & Quantum Numbers49:32
34m 4s
- Intro0:00
- Electron Configurations & Diagrams1:08
- Electronic Structure of Ground State Atom1:09
- Order of Electron Filling3:50
- Electron Configurations & Diagrams: H8:41
- Electron Configurations & Diagrams: He9:12
- Electron Configurations & Diagrams: Li9:47
- Electron Configurations & Diagrams: Be11:17
- Electron Configurations & Diagrams: B12:05
- Electron Configurations & Diagrams: C13:03
- Electron Configurations & Diagrams: N14:55
- Electron Configurations & Diagrams: O15:24
- Electron Configurations & Diagrams: F16:25
- Electron Configurations & Diagrams: Ne17:00
- Electron Configurations & Diagrams: S18:08
- Electron Configurations & Diagrams: Fe20:08
- Introduction to Valence Electrons23:04
- Valence Electrons of Oxygen23:44
- Valence Electrons of Iron24:02
- Valence Electrons of Arsenic24:30
- Valence Electrons: Exceptions25:36
- The Periodic Table27:52
52m 43s
- Intro0:00
- Vapor Pressure and Changes of State2:26
- Intermolecular Forces Overview2:27
- Hydrogen Bonding5:23
- Heat of Vaporization9:58
- Vapor Pressure: Definition and Example11:04
- Vapor Pressures is Mostly a Function of Intermolecular Forces17:41
- Vapor Pressure Increases with Temperature20:52
- Vapor Pressure vs. Temperature: Graph and Equation22:55
- Clausius-Clapeyron Equation31:55
- Example 132:13
- Heating Curve35:40
- Heat of Fusion41:31
- Example 243:45
31m 17s
- Intro0:00
- Phase Diagrams and Solutions0:22
- Definition of a Phase Diagram0:50
- Phase Diagram Part 1: H₂O1:54
- Phase Diagram Part 2: CO₂9:59
- Solutions: Solute & Solvent16:12
- Ways of Discussing Solution Composition: Mass Percent or Weight Percent18:46
- Ways of Discussing Solution Composition: Molarity20:07
- Ways of Discussing Solution Composition: Mole Fraction20:48
- Ways of Discussing Solution Composition: Molality21:41
- Example 1: Question22:06
- Example 1: Mass Percent24:32
- Example 1: Molarity25:53
- Example 1: Mole Fraction28:09
- Example 1: Molality29:36
37m 23s
- Intro0:00
- Vapor Pressure of Solutions2:07
- Vapor Pressure & Raoult's Law2:08
- Example 15:21
- When Ionic Compounds Dissolve10:51
- Example 212:38
- Non-Ideal Solutions17:42
- Negative Deviation24:23
- Positive Deviation29:19
- Example 331:40
34m 11s
- Intro0:00
- Colligative Properties1:07
- Boiling Point Elevation1:08
- Example 1: Question5:19
- Example 1: Solution6:52
- Freezing Point Depression12:01
- Example 2: Question14:46
- Example 2: Solution16:34
- Osmotic Pressure20:20
- Example 3: Question28:00
- Example 3: Solution30:16
48m 39s
- Intro0:00
- Bonding & Lewis Structure2:23
- Covalent Bond2:24
- Single Bond, Double Bond, and Triple Bond4:11
- Bond Length & Intermolecular Distance5:51
- Definition of Electronegativity8:42
- Bond Polarity11:48
- Bond Energy20:04
- Example 124:31
- Definition of Lewis Structure31:54
- Steps in Forming a Lewis Structure33:26
- Lewis Structure Example: H₂36:53
- Lewis Structure Example: CH₄37:33
- Lewis Structure Example: NO⁺38:43
- Lewis Structure Example: PCl₅41:12
- Lewis Structure Example: ICl₄⁻43:05
- Lewis Structure Example: BeCl₂45:07
36m 59s
- Intro0:00
- Resonance and Formal Charge0:09
- Resonance Structures of NO₃⁻0:25
- Resonance Structures of NO₂⁻12:28
- Resonance Structures of HCO₂⁻16:28
- Formal Charge19:40
- Formal Charge Example: SO₄²⁻21:32
- Formal Charge Example: CO₂31:33
- Formal Charge Example: HCN32:44
- Formal Charge Example: CN⁻33:34
- Formal Charge Example: 0₃34:43
41m 21s
- Intro0:00
- Shapes of Molecules0:35
- VSEPR0:36
- Steps in Determining Shapes of Molecules6:18
- Linear11:38
- Trigonal Planar11:55
- Tetrahedral12:45
- Trigonal Bipyramidal13:23
- Octahedral14:29
- Table: Shapes of Molecules15:40
- Example: CO₂21:11
- Example: NO₃⁻24:01
- Example: H₂O27:00
- Example: NH₃29:48
- Example: PCl₃⁻32:18
- Example: IF₄⁺34:38
- Example: KrF₄37:57
40m 17s
- Intro0:00
- Hybrid Orbitals0:13
- Introduction to Hybrid Orbitals0:14
- Electron Orbitals for CH₄5:02
- sp³ Hybridization10:52
- Example: sp³ Hybridization12:06
- sp² Hybridization14:21
- Example: sp² Hybridization16:11
- σ Bond19:10
- π Bond20:07
- sp Hybridization & Example22:00
- dsp³ Hybridization & Example27:36
- d²sp³ Hybridization & Example30:36
- Example: Predict the Hybridization and Describe the Molecular Geometry of CO32:31
- Example: Predict the Hybridization and Describe the Molecular Geometry of BF₄⁻35:17
- Example: Predict the Hybridization and Describe the Molecular Geometry of XeF₂37:09
52m 34s
- Intro0:00
- Multiple Choice1:21
- Multiple Choice 11:22
- Multiple Choice 22:23
- Multiple Choice 33:38
- Multiple Choice 44:34
- Multiple Choice 55:16
- Multiple Choice 65:41
- Multiple Choice 76:20
- Multiple Choice 87:03
- Multiple Choice 97:31
- Multiple Choice 109:03
- Multiple Choice 1111:52
- Multiple Choice 1213:16
- Multiple Choice 1313:56
- Multiple Choice 1414:52
- Multiple Choice 1515:43
- Multiple Choice 1616:20
- Multiple Choice 1716:55
- Multiple Choice 1817:22
- Multiple Choice 1918:59
- Multiple Choice 2020:24
- Multiple Choice 2122:20
- Multiple Choice 2223:29
- Multiple Choice 2324:30
- Multiple Choice 2425:24
- Multiple Choice 2526:21
- Multiple Choice 2629:06
- Multiple Choice 2730:42
- Multiple Choice 2833:28
- Multiple Choice 2934:38
- Multiple Choice 3035:37
- Multiple Choice 3137:31
- Multiple Choice 3238:28
- Multiple Choice 3339:50
- Multiple Choice 3442:57
- Multiple Choice 3544:18
- Multiple Choice 3645:52
- Multiple Choice 3748:02
- Multiple Choice 3849:25
- Multiple Choice 3949:43
- Multiple Choice 4050:16
- Multiple Choice 4150:49
32m 15s
- Intro0:00
- Multiple Choice0:12
- Multiple Choice 420:13
- Multiple Choice 430:33
- Multiple Choice 441:16
- Multiple Choice 452:36
- Multiple Choice 465:22
- Multiple Choice 476:35
- Multiple Choice 488:02
- Multiple Choice 4910:05
- Multiple Choice 5010:26
- Multiple Choice 5111:07
- Multiple Choice 5212:01
- Multiple Choice 5312:55
- Multiple Choice 5416:12
- Multiple Choice 5518:11
- Multiple Choice 5619:45
- Multiple Choice 5720:15
- Multiple Choice 5823:28
- Multiple Choice 5924:27
- Multiple Choice 6026:45
- Multiple Choice 6129:15
32m 50s
- Intro0:00
- Multiple Choice0:16
- Multiple Choice 620:17
- Multiple Choice 631:57
- Multiple Choice 646:16
- Multiple Choice 658:05
- Multiple Choice 669:18
- Multiple Choice 6710:38
- Multiple Choice 6812:51
- Multiple Choice 6914:32
- Multiple Choice 7017:35
- Multiple Choice 7122:44
- Multiple Choice 7224:27
- Multiple Choice 7327:46
- Multiple Choice 7429:39
- Multiple Choice 7530:23
47m 22s
- Intro0:00
- Free Response0:15
- Free Response 1: Part A0:16
- Free Response 1: Part B4:15
- Free Response 1: Part C5:47
- Free Response 1: Part D9:20
- Free Response 1: Part E. i10:58
- Free Response 1: Part E. ii16:45
- Free Response 1: Part E. iii26:03
- Free Response 2: Part A. i31:01
- Free Response 2: Part A. ii33:38
- Free Response 2: Part A. iii35:20
- Free Response 2: Part B. i37:38
- Free Response 2: Part B. ii39:30
- Free Response 2: Part B. iii44:44
43m 5s
- Intro0:00
- Free Response0:12
- Free Response 3: Part A0:13
- Free Response 3: Part B6:25
- Free Response 3: Part C. i11:33
- Free Response 3: Part C. ii12:02
- Free Response 3: Part D14:30
- Free Response 4: Part A21:03
- Free Response 4: Part B22:59
- Free Response 4: Part C24:33
- Free Response 4: Part D27:22
- Free Response 4: Part E28:43
- Free Response 4: Part F29:35
- Free Response 4: Part G30:15
- Free Response 4: Part H30:48
- Free Response 5: Diagram32:00
- Free Response 5: Part A34:14
- Free Response 5: Part B36:07
- Free Response 5: Part C37:45
- Free Response 5: Part D39:00
- Free Response 5: Part E40:26
28m 36s
- Intro0:00
- Free Response0:43
- Free Response 6: Part A. i0:44
- Free Response 6: Part A. ii3:08
- Free Response 6: Part A. iii5:02
- Free Response 6: Part B. i7:11
- Free Response 6: Part B. ii9:40
- Free Response 7: Part A11:14
- Free Response 7: Part B13:45
- Free Response 7: Part C15:43
- Free Response 7: Part D16:54
- Free Response 8: Part A. i19:15
- Free Response 8: Part A. ii21:16
- Free Response 8: Part B. i23:51
- Free Response 8: Part B. ii25:07
For more information, please see full course syllabus of AP Chemistry
AP Chemistry Energy, Heat, and Work
Thermochemistry is chemistry related to heat—which is not the same thing as temperature! Temperature is the average kinetic energy (random movement) of particles in a sample. Heat is the energy flows between parts of a sample when they are at different temperatures (it flows from “hot” to “cold”). Thermochemistry is about the conversion of energy from heat to other forms like work (force times distance in units of Newton-meters or Joules). Thermochemistry problems usually involve a “system” and the “surroundings.” Endothermic reactions transfer heat from the surroundings to the system. Exothermic reactions give off heat, transferring it from the system to the surroundings. When work is being done on the system by the surroundings, work has a positive sign. It is negative when the system does work on the surroundings.
Share this knowledge with your friends!
Copy & Paste this embed code into your website’s HTML
Please ensure that your website editor is in text mode when you paste the code.(In Wordpress, the mode button is on the top right corner.)
- - Allow users to view the embedded video in full-size.
1 answer
Tue Jun 23, 2020 3:17 PM
Post by Grace He on June 23, 2020
Hi professor Hovasapian!
Concerning the change in energy formula, if dE=q+w; and w=-PdV; why would it be w=q+PdV and not w=q-PdV since w=-PdV?
1 answer
Tue Nov 20, 2018 4:07 AM
Post by Holden Kim on November 19, 2018
Hello,
For the equation: Work = - Pressure x deltaVolume
Does the pressure refer to the pressure of the gas inside of the chamber?
2 answers
Last reply by: Holden Kim
Mon Nov 19, 2018 10:55 PM
Post by Holden Kim on November 19, 2018
At 32:52, why did you attach a negative symbol to P?V?
2 answers
Wed Jan 18, 2017 6:15 PM
Post by Magic Fu on December 20, 2016
Hi Professor H
I think you balanced your equation wrong at 13:23. You had 2 carbons on the product side while only one on the reactant side. You had six oxygens on the product while only 4 on the reactant side.
1 answer
Sun Oct 30, 2016 5:18 PM
Post by Warwick Shaw on October 30, 2016
Hi,
If delta E equals q + w or as you put it, q + p times delta v, wouldn't that mean that delta E is equal to q + negative w ( because work is negative p times delta v and just p times delta v would be negative work)?
3 answers
Last reply by: Jason Smith
Sun Sep 27, 2015 3:54 PM
Post by Jason Smith on September 26, 2015
Hi professor. If I understand correctly, "heat" doesn't necessarily mean "hot", right? It basically depends on the difference in temperature between two objects- is this correct? Thank you in advance.
1 answer
Sun Sep 27, 2015 1:20 AM
Post by Gaurav Kumar on September 26, 2015
Hi Professor Hovasapain,
I am confused to why N2 + O2 ----> 2NO is a endothermic process. Can you please explain?
Thank you
1 answer
Sat Aug 8, 2015 9:49 PM
Post by Jim Tang on August 7, 2015
Hey!
31:15 shouldn't that be negative?
Also, in example 3, the pressure that the balloon expands AGAINST is the pressure the surroundings is pushing ON the balloon correct?
Ty!
1 answer
Sat Sep 6, 2014 11:10 PM
Post by David Gonzalez on August 31, 2014
Hi profession, great lecture. You are such an amazing teacher!
Just to help me wrap my head around this, please tell me if the analogy that I have in my head is correct: if you place a drop of food coloring into a glass of water, it will "disperse" until it reaches equilibrium. Does this mean that the heat particles/molecules are trying to do the same thing - "disperse" to achieve equilibrium?
Thanks!
1 answer
Wed Jul 9, 2014 6:41 PM
Post by ibrahim shawi on July 8, 2014
hello professor Hovasapain, my question here is about work&gas you said the work done by the gas on the system (expansion) gives us w=-p(deltaV) and i get that because earlier in the video you explained work or energy leaving the system is negative and it is positive if it is entering the system, what im confused on is in my mcat prep book it says "this equation (w=p(deltaV)) *(constant pressure) shows the work done by the gas. the work done on the gas is just the negative of the work done by the gas, so you might see tis equation written with a negative sign as (w=-p(deltaV)). why do you think this may be? and also how can pressure be constant if it is inversely proportional to V. like if volume was to increase pressure would decrease. do the mean the more gas is pumped in to keep the pressure constant?
Thank you.
1 answer
Wed May 15, 2013 2:04 AM
Post by Nawaphan Jedjomnongkit on May 14, 2013
Thank you for the lecture but I tend to have problem when try to link things up. What I've learnt from here is that when we do work ON a system , the volume will decrease and energy of the system will gain, right? But from the relationship of volume and temp from ideal gas law is in the way that when we decrease volume it will decrease temperature. And when I relate temperature to KE of the system it will be decrease, so is that mean when the system gain energy, their KE will decrease and where the energy gone?
1 answer
Fri Apr 26, 2013 8:28 PM
Post by Shaurntae Thomas on April 26, 2013
Why was the answer 1330.069 J and not -1330.069 J? This question comes from the work equals pressure and volume equation.
1 answer
Sat Apr 20, 2013 6:10 PM
Post by Antie Chen on April 20, 2013
Wï¼P(delta)V
The equation is from W=Force*Distance=P(delta)V
The unit of P is atm and the Unit of V is m3, 1N*m=1j why 1 atm*m3 is not equal to 1 joule but need to use 1 atm*m3=101.3j to convert?
1 answer
Fri Feb 1, 2013 3:17 AM
Post by Gayatri Arumugam on January 31, 2013
Hi Professor Hovasapain,
In Example two why is the answer 1330.04 J instead of -1330.07 J?
Thanks,
Gayatri Aruumugam
1 answer
Wed Jan 16, 2013 9:02 PM
Post by Stephen Piesley on January 16, 2013
If the equation format is
(delta) E = q + w.
The Last part of the equation Example III
is set out as :
(q) 1.4 x 10 power of 8 + (w) -4.8 x 10 power of 7
Had did this equate to the answer 9.2 x 10 power of 7 ,Have I missed something in the Arithmetic.?
1 answer
Wed Nov 28, 2012 2:02 PM
Post by Julie Mohamed on November 26, 2012
How would i find the temperature of hot water with a thermometer that only reads to 50 degrees Celsius?
Supplies : styrofoam cup
50 degree thermometer
tap water
Hot water
Electronic balance
0 answers
Post by Themiya Chandraratna on May 6, 2012
v. is for volume
1 answer
Sat Jul 14, 2012 6:31 PM
Post by Miguel Ibanez on March 24, 2012
can you explain the W=P(delta)V formula? does "v" mean the change in area of the balloon?